Ovarian cancer modulates the immunosuppressive function of CD11b+Gr1+ myeloid cells via glutamine metabolism
- PMID: 34144215
- PMCID: PMC8267600
- DOI: 10.1016/j.molmet.2021.101272
Ovarian cancer modulates the immunosuppressive function of CD11b+Gr1+ myeloid cells via glutamine metabolism
Abstract
Objective: Immature CD11b + Gr1+ myeloid cells that acquire immunosuppressive capability, also known as myeloid-derived suppressor cells (MDSCs), are a heterogeneous population of cells that regulate immune responses. Our study's objective was to elucidate the role of ovarian cancer microenvironment in regulating the immunosuppressive function of CD11b+Gr1+ myeloid cells.
Methods: All studies were performed using the intraperitoneal ID8 syngeneic epithelial ovarian cancer mouse model. Myeloid cell depletion and immunotherapy were carried out using anti-Gr1 mAb, gemcitabine treatments, and/or anti-PD1 mAb. The treatment effect was assessed by a survival curve, in situ luciferase-guided imaging, and histopathologic evaluation. Adoptive transfer assays were carried out between congenic CD45.2 and CD45.1 mice. Immune surface and intracellular markers were assessed by flow cytometry. ELISA, western blot, and RT-PCR techniques were employed to assess the protein and RNA expression of various markers. Bone marrow-derived myeloid cells were used for ex-vivo studies.
Results: The depletion of Gr1+ immunosuppressive myeloid cells alone and in combination with anti-PD1 immunotherapy inhibited ovarian cancer growth. In addition to the adoptive transfer studies, these findings validate the role of immunosuppressive CD11b+Gr1+ myeloid cells in promoting ovarian cancer. Mechanistic investigations showed that ID8 tumor cells and their microenvironments produced recruitment and regulatory factors for immunosuppressive CD11b+Gr1+ myeloid cells. CD11b+Gr1+ myeloid cells primed by ID8 tumors showed increased immunosuppressive marker expression and acquired an energetic metabolic phenotype promoted primarily by increased oxidative phosphorylation fueled by glutamine. Inhibiting the glutamine metabolic pathway reduced the increased oxidative phosphorylation and decreased immunosuppressive markers' expression and function. Dihydrolipoamide succinyl transferase (DLST), a subunit of α-KGDC in the TCA cycle, was found to be the most significantly elevated gene in tumor-primed myeloid cells. The inhibition of DLST reduced oxidative phosphorylation, immunosuppressive marker expression and function in myeloid cells.
Conclusion: Our study shows that the ovarian cancer microenvironment can regulate the metabolism and function of immunosuppressive CD11b + Gr1+ myeloid cells and modulate its immune microenvironment. Targeting glutamine metabolism via DLST in immunosuppressive myeloid cells decreased their activity, leading to a reduction in the immunosuppressive tumor microenvironment. Thus, targeting glutamine metabolism has the potential to enhance the success of immunotherapy in ovarian cancer.
Keywords: CD11b(+)Gr1(+) immunosuppressive cells; DLST; Glutamine metabolism; MDSCs; Ovarian cancer; α-KGDC.
Copyright © 2021 The Author(s). Published by Elsevier GmbH.. All rights reserved.
Figures
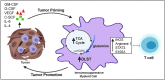
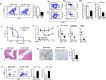
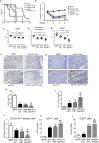
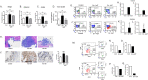
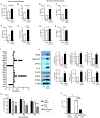
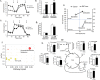
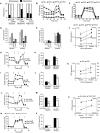
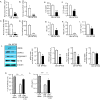
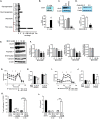
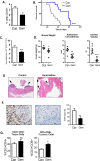
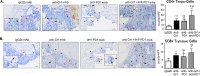
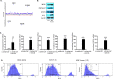
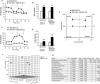
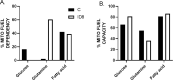
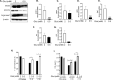
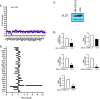
Similar articles
-
Microsomal prostaglandin E synthase-1 promotes lung metastasis via SDF-1/CXCR4-mediated recruitment of CD11b+Gr1+MDSCs from bone marrow.Biomed Pharmacother. 2020 Jan;121:109581. doi: 10.1016/j.biopha.2019.109581. Epub 2019 Nov 10. Biomed Pharmacother. 2020. PMID: 31715374
-
Tumor refractoriness to endostatin anti-angiogenesis is associated with the recruitment of CD11b+Gr1+ myeloid cells and inflammatory cytokines.Tumori. 2013 Nov-Dec;99(6):723-33. doi: 10.1177/030089161309900613. Tumori. 2013. PMID: 24503797
-
Targeting myeloid cells in the tumor microenvironment enhances vaccine efficacy in murine epithelial ovarian cancer.Oncotarget. 2015 May 10;6(13):11310-26. doi: 10.18632/oncotarget.3597. Oncotarget. 2015. PMID: 25888637 Free PMC article.
-
The role of CCR5 in directing the mobilization and biological function of CD11b+Gr1+Ly6Clow polymorphonuclear myeloid cells in cancer.Cancer Immunol Immunother. 2018 Dec;67(12):1949-1953. doi: 10.1007/s00262-018-2245-6. Epub 2018 Sep 19. Cancer Immunol Immunother. 2018. PMID: 30232521 Free PMC article. Review.
-
LPS-induced CD11b+Gr1(int)F4/80+ regulatory myeloid cells suppress allergen-induced airway inflammation.Int Immunopharmacol. 2011 Jul;11(7):827-32. doi: 10.1016/j.intimp.2011.01.034. Epub 2011 Feb 12. Int Immunopharmacol. 2011. PMID: 21320637 Free PMC article. Review.
Cited by
-
Comprehensive analysis of cuproptosis-related lncRNAs model in tumor immune microenvironment and prognostic value of cervical cancer.Front Pharmacol. 2022 Nov 30;13:1065701. doi: 10.3389/fphar.2022.1065701. eCollection 2022. Front Pharmacol. 2022. PMID: 36532719 Free PMC article.
-
Combining all-trans retinoid acid treatment targeting myeloid-derived suppressive cells with cryo-thermal therapy enhances antitumor immunity in breast cancer.Front Immunol. 2022 Nov 1;13:1016776. doi: 10.3389/fimmu.2022.1016776. eCollection 2022. Front Immunol. 2022. PMID: 36389684 Free PMC article.
-
Identification of an Amino Acid Metabolism Signature Participating in Immunosuppression in Ovarian Cancer.Evid Based Complement Alternat Med. 2022 Jun 22;2022:4525540. doi: 10.1155/2022/4525540. eCollection 2022. Evid Based Complement Alternat Med. 2022. PMID: 35783506 Free PMC article.
-
Harnessing innate immune pathways for therapeutic advancement in cancer.Signal Transduct Target Ther. 2024 Mar 25;9(1):68. doi: 10.1038/s41392-024-01765-9. Signal Transduct Target Ther. 2024. PMID: 38523155 Free PMC article. Review.
-
Identification and validation of novel genes related to immune microenvironment in polycystic ovary syndrome.Medicine (Baltimore). 2024 Oct 25;103(43):e40229. doi: 10.1097/MD.0000000000040229. Medicine (Baltimore). 2024. PMID: 39470566 Free PMC article.
References
-
- Bogani G., Lopez S., Mantiero M., Ducceschi M., Bosio S., Ruisi S. Immunotherapy for platinum-resistant ovarian cancer. Gynecologic Oncology. 2020;158(2):484–488. - PubMed
-
- Odunsi K., Jungbluth A.A., Stockert E., Qian F., Gnjatic S., Tammela J. NY-ESO-1 and LAGE-1 cancer-testis antigens are potential targets for immunotherapy in epithelial ovarian cancer. Cancer Research. 2003;63(18):6076–6083. - PubMed
Publication types
MeSH terms
Substances
Grants and funding
LinkOut - more resources
Full Text Sources
Medical
Research Materials
Miscellaneous