Cell Membrane Coating Technology: A Promising Strategy for Biomedical Applications
- PMID: 34138027
- PMCID: PMC7770915
- DOI: 10.1007/s40820-019-0330-9
Cell Membrane Coating Technology: A Promising Strategy for Biomedical Applications
Abstract
Cell membrane coating technology is an approach to the biomimetic replication of cell membrane properties, and is an active area of ongoing research readily applicable to nanoscale biomedicine. Nanoparticles (NPs) coated with cell membranes offer an opportunity to unite natural cell membrane properties with those of the artificial inner core material. The coated NPs not only increase their biocompatibility but also achieve effective and extended circulation in vivo, allowing for the execution of targeted functions. Although cell membrane-coated NPs offer clear advantages, much work remains before they can be applied in clinical practice. In this review, we first provide a comprehensive overview of the theory of cell membrane coating technology, followed by a summary of the existing preparation and characterization techniques. Next, we focus on the functions and applications of various cell membrane types. In addition, we collate model drugs used in cell membrane coating technology, and review the patent applications related to this technology from the past 10 years. Finally, we survey future challenges and trends pertaining to this technology in an effort to provide a comprehensive overview of the future development of cell membrane coating technology.
Keywords: Biomimetic nanoparticles; Cancer therapy; Cell membrane; Detoxification; Immune modulation.
Figures
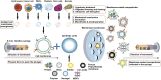
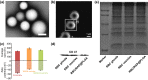
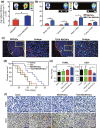
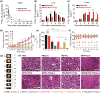
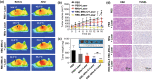
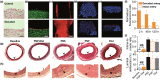
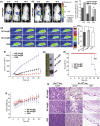
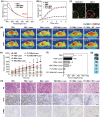
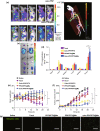
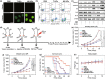
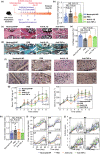
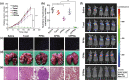
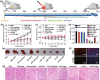
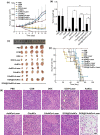
Similar articles
-
Cellular Membrane Components-Mediated Cancer Immunotherapeutic Platforms.Macromol Biosci. 2023 Nov;23(11):e2300159. doi: 10.1002/mabi.202300159. Epub 2023 Jun 25. Macromol Biosci. 2023. PMID: 37319369 Review.
-
Biomimetic Nanoparticles Coated with Bacterial Outer Membrane Vesicles as a New-Generation Platform for Biomedical Applications.Pharmaceutics. 2021 Nov 7;13(11):1887. doi: 10.3390/pharmaceutics13111887. Pharmaceutics. 2021. PMID: 34834302 Free PMC article. Review.
-
Hybrid Membrane-Coated Biomimetic Nanoparticles (HM@BNPs): A Multifunctional Nanomaterial for Biomedical Applications.Biomacromolecules. 2021 Aug 9;22(8):3149-3167. doi: 10.1021/acs.biomac.1c00440. Epub 2021 Jul 6. Biomacromolecules. 2021. PMID: 34225451 Review.
-
Surface modification potentials of cell membrane-based materials for targeted therapies: a chemotherapy-focused review.Nanomedicine (Lond). 2023 Aug;18(19):1281-1303. doi: 10.2217/nnm-2023-0164. Epub 2023 Sep 27. Nanomedicine (Lond). 2023. PMID: 37753724 Review.
-
Erythrocytes Nanoparticle Delivery: A Boon for Targeting Tumor.Adv Pharm Bull. 2024 Mar;14(1):132-146. doi: 10.34172/apb.2023.080. Epub 2023 Apr 29. Adv Pharm Bull. 2024. PMID: 38585450 Free PMC article. Review.
Cited by
-
Biomimetic Nanocarriers for Cancer Target Therapy.Bioengineering (Basel). 2020 Sep 14;7(3):111. doi: 10.3390/bioengineering7030111. Bioengineering (Basel). 2020. PMID: 32937963 Free PMC article. Review.
-
Biomimetic cell membrane-coated poly(lactic-co-glycolic acid) nanoparticles for biomedical applications.Bioeng Transl Med. 2022 Nov 2;8(2):e10441. doi: 10.1002/btm2.10441. eCollection 2023 Mar. Bioeng Transl Med. 2022. PMID: 36925703 Free PMC article. Review.
-
Panax Notoginseng Saponins Regulate Transforming Growth Factor-β1 through MAPK and Snail/TWIST1 Signaling Pathway to Inhibit Epithelial-Mesenchymal Transition of Pulmonary Fibrosis in A549 Cells.Evid Based Complement Alternat Med. 2022 Jul 12;2022:3744618. doi: 10.1155/2022/3744618. eCollection 2022. Evid Based Complement Alternat Med. 2022. PMID: 35865337 Free PMC article.
-
Superparamagnetic Iron Oxide Nanoparticles: Cytotoxicity, Metabolism, and Cellular Behavior in Biomedicine Applications.Int J Nanomedicine. 2021 Aug 31;16:6097-6113. doi: 10.2147/IJN.S321984. eCollection 2021. Int J Nanomedicine. 2021. PMID: 34511908 Free PMC article. Review.
-
T Cell and Natural Killer Cell Membrane-Camouflaged Nanoparticles for Cancer and Viral Therapies.ACS Appl Bio Mater. 2024 May 20;7(5):2637-2659. doi: 10.1021/acsabm.4c00074. Epub 2024 Apr 30. ACS Appl Bio Mater. 2024. PMID: 38687958 Free PMC article. Review.
References
Publication types
LinkOut - more resources
Full Text Sources
Other Literature Sources
Miscellaneous