Metabolically engineered stem cell-derived exosomes to regulate macrophage heterogeneity in rheumatoid arthritis
- PMID: 34078596
- PMCID: PMC8172131
- DOI: 10.1126/sciadv.abe0083
Metabolically engineered stem cell-derived exosomes to regulate macrophage heterogeneity in rheumatoid arthritis
Abstract
Despite the remarkable advances in therapeutics for rheumatoid arthritis (RA), a large number of patients still lack effective countermeasures. Recently, the reprogramming of macrophages to an immunoregulatory phenotype has emerged as a promising therapeutic strategy for RA. Here, we report metabolically engineered exosomes that have been surface-modified for the targeted reprogramming of macrophages. Qualified exosomes were readily harvested from metabolically engineered stem cells by tangential flow filtration at a high yield while maintaining their innate immunomodulatory components. When systemically administered into mice with collagen-induced arthritis, these exosomes effectively accumulated in the inflamed joints, inducing a cascade of anti-inflammatory events via macrophage phenotype regulation. The level of therapeutic efficacy obtained with bare exosomes was achievable with the engineered exosomes of 10 times less dose. On the basis of the boosted nature to reprogram the synovial microenvironment, the engineered exosomes display considerable potential to be developed as a next-generation drug for RA.
Copyright © 2021 The Authors, some rights reserved; exclusive licensee American Association for the Advancement of Science. No claim to original U.S. Government Works. Distributed under a Creative Commons Attribution NonCommercial License 4.0 (CC BY-NC).
Figures
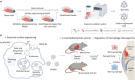
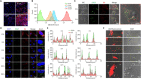
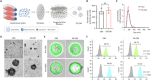
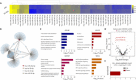
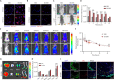
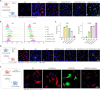
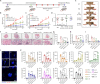
Comment in
-
Modifying exosomes to target macrophages in arthritis.Nat Rev Rheumatol. 2021 Aug;17(8):443. doi: 10.1038/s41584-021-00651-w. Nat Rev Rheumatol. 2021. PMID: 34188204 No abstract available.
Similar articles
-
Reactive oxygen species-responsive dendritic cell-derived exosomes for rheumatoid arthritis.Acta Biomater. 2021 Jul 1;128:462-473. doi: 10.1016/j.actbio.2021.04.026. Epub 2021 Apr 18. Acta Biomater. 2021. PMID: 33878476
-
Therapeutic Potential of Mesenchymal Cell-Derived miRNA-150-5p-Expressing Exosomes in Rheumatoid Arthritis Mediated by the Modulation of MMP14 and VEGF.J Immunol. 2018 Oct 15;201(8):2472-2482. doi: 10.4049/jimmunol.1800304. Epub 2018 Sep 17. J Immunol. 2018. PMID: 30224512 Free PMC article.
-
A Targeted Exosome Therapeutic Confers Both CfDNA Scavenging and Macrophage Polarization for Ameliorating Rheumatoid Arthritis.Adv Mater. 2023 Nov;35(48):e2302503. doi: 10.1002/adma.202302503. Epub 2023 Oct 27. Adv Mater. 2023. PMID: 37681753
-
Exploring the role of exosomes in rheumatoid arthritis.Inflammopharmacology. 2023 Feb;31(1):119-128. doi: 10.1007/s10787-022-01100-0. Epub 2022 Nov 21. Inflammopharmacology. 2023. PMID: 36414831 Review.
-
Stem Cell Mimicking Nanoencapsulation for Targeting Arthritis.Int J Nanomedicine. 2021 Dec 31;16:8485-8507. doi: 10.2147/IJN.S334298. eCollection 2021. Int J Nanomedicine. 2021. PMID: 35002240 Free PMC article. Review.
Cited by
-
Preparation of genetically or chemically engineered exosomes and their therapeutic effects in bone regeneration and anti-inflammation.Front Bioeng Biotechnol. 2024 Jan 19;12:1329388. doi: 10.3389/fbioe.2024.1329388. eCollection 2024. Front Bioeng Biotechnol. 2024. PMID: 38314353 Free PMC article. Review.
-
Small extracellular vesicles derived from hypoxic preconditioned dental pulp stem cells ameliorate inflammatory osteolysis by modulating macrophage polarization and osteoclastogenesis.Bioact Mater. 2022 Oct 19;22:326-342. doi: 10.1016/j.bioactmat.2022.10.001. eCollection 2023 Apr. Bioact Mater. 2022. PMID: 36311048 Free PMC article.
-
Mesenchymal Stem Cell-Derived Exosomes: Applications in Regenerative Medicine.Cells. 2021 Aug 1;10(8):1959. doi: 10.3390/cells10081959. Cells. 2021. PMID: 34440728 Free PMC article. Review.
-
Mesenchymal stem cell-derived extracellular vesicles in joint diseases: Therapeutic effects and underlying mechanisms.J Orthop Translat. 2024 Jul 27;48:53-69. doi: 10.1016/j.jot.2024.07.005. eCollection 2024 Sep. J Orthop Translat. 2024. PMID: 39170747 Free PMC article. Review.
-
Inflammation in Fabry disease: stages, molecular pathways, and therapeutic implications.Front Cardiovasc Med. 2024 Jun 12;11:1420067. doi: 10.3389/fcvm.2024.1420067. eCollection 2024. Front Cardiovasc Med. 2024. PMID: 38932991 Free PMC article. Review.
References
-
- Olumuyiwa-Akeredolu O.-o., Page M. J., Soma P., Pretorius E., Platelets: Emerging facilitators of cellular crosstalk in rheumatoid arthritis. Nat. Rev. Rheumatol. 15, 237–248 (2019). - PubMed
-
- Smolen J. S., Steiner G., Therapeutic strategies for rheumatoid arthritis. Nat. Rev. Drug Discov. 2, 473–488 (2003). - PubMed
-
- Fortune Business Insights (2019); www.fortunebusinessinsights.com/industry-reports/rheumatoid-arthritis-th....
-
- Smolen J. S., Aletaha D., Barton A., Burmester G. R., Emery P., Firestein G. S., Kavanaugh A., McInnes I. B., Solomon D. H., Strand V., Yamamoto K., Rheumatoid arthritis. Nat. Rev. Dis. Primers. 4, 18001 (2018). - PubMed
-
- Udalova I. A., Mantovani A., Feldmann M., Macrophage heterogeneity in the context of rheumatoid arthritis. Nat. Rev. Rheumatol. 12, 472–485 (2016). - PubMed
Publication types
MeSH terms
LinkOut - more resources
Full Text Sources
Medical