Cross-reactive serum and memory B-cell responses to spike protein in SARS-CoV-2 and endemic coronavirus infection
- PMID: 34011939
- PMCID: PMC8134462
- DOI: 10.1038/s41467-021-23074-3
Cross-reactive serum and memory B-cell responses to spike protein in SARS-CoV-2 and endemic coronavirus infection
Abstract
Pre-existing immunity to seasonal endemic coronaviruses could have profound consequences for antibody responses to SARS-CoV-2, induced from natural infection or vaccination. A first step to establish whether pre-existing responses can impact SARS-CoV-2 infection is to understand the nature and extent of cross-reactivity in humans to coronaviruses. Here we compare serum antibody and memory B cell responses to coronavirus spike proteins from pre-pandemic and SARS-CoV-2 convalescent donors using binding and functional assays. We show weak evidence of pre-existing SARS-CoV-2 cross-reactive serum antibodies in pre-pandemic donors. However, we find evidence of pre-existing cross-reactive memory B cells that are activated during SARS-CoV-2 infection. Monoclonal antibodies show varying degrees of cross-reactivity with betacoronaviruses, including SARS-CoV-1 and endemic coronaviruses. We identify one cross-reactive neutralizing antibody specific to the S2 subunit of the S protein. Our results suggest that pre-existing immunity to endemic coronaviruses should be considered in evaluating antibody responses to SARS-CoV-2.
Conflict of interest statement
R.A., G.S., W.H., T.F.R., and D.R.B. are listed as inventors on pending patent applications describing the SARS-CoV-2 and HCoV-HKU1 S cross-reactive antibodies. D.R.B. is a consultant for IAVI. All other authors have no competing interests to declare.
Figures
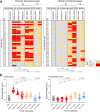
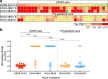
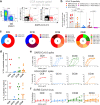
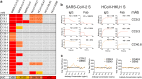
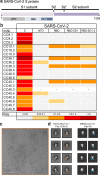
Update of
-
Cross-reactive serum and memory B cell responses to spike protein in SARS-CoV-2 and endemic coronavirus infection.bioRxiv [Preprint]. 2020 Sep 23:2020.09.22.308965. doi: 10.1101/2020.09.22.308965. bioRxiv. 2020. Update in: Nat Commun. 2021 May 19;12(1):2938. doi: 10.1038/s41467-021-23074-3. PMID: 32995770 Free PMC article. Updated. Preprint.
Similar articles
-
Cross-reactive serum and memory B cell responses to spike protein in SARS-CoV-2 and endemic coronavirus infection.bioRxiv [Preprint]. 2020 Sep 23:2020.09.22.308965. doi: 10.1101/2020.09.22.308965. bioRxiv. 2020. Update in: Nat Commun. 2021 May 19;12(1):2938. doi: 10.1038/s41467-021-23074-3. PMID: 32995770 Free PMC article. Updated. Preprint.
-
S Protein-Reactive IgG and Memory B Cell Production after Human SARS-CoV-2 Infection Includes Broad Reactivity to the S2 Subunit.mBio. 2020 Sep 25;11(5):e01991-20. doi: 10.1128/mBio.01991-20. mBio. 2020. PMID: 32978311 Free PMC article.
-
Non-cross-reactive epitopes dominate the humoral immune response to COVID-19 vaccination - kinetics of plasma antibodies, plasmablasts and memory B cells.Front Immunol. 2024 May 14;15:1382911. doi: 10.3389/fimmu.2024.1382911. eCollection 2024. Front Immunol. 2024. PMID: 38807606 Free PMC article.
-
Antibody and B cell responses to SARS-CoV-2 infection and vaccination.Cell Host Microbe. 2021 Jul 14;29(7):1063-1075. doi: 10.1016/j.chom.2021.06.009. Epub 2021 Jun 17. Cell Host Microbe. 2021. PMID: 34174992 Free PMC article. Review.
-
Heterologous humoral immunity to human and zoonotic coronaviruses: Aiming for the achilles heel.Semin Immunol. 2021 Jun;55:101507. doi: 10.1016/j.smim.2021.101507. Epub 2021 Oct 25. Semin Immunol. 2021. PMID: 34716096 Free PMC article. Review.
Cited by
-
Antibody Responses in SARS-CoV-2-Exposed and/or Vaccinated Individuals Target Conserved Epitopes from Multiple CoV-2 Antigens.Int J Mol Sci. 2024 Sep 11;25(18):9814. doi: 10.3390/ijms25189814. Int J Mol Sci. 2024. PMID: 39337303 Free PMC article.
-
Ultrafast and high-throughput immunoassay assay to detect anti-SARS-CoV-2 IgG antibodies in dogs and cats.Braz J Microbiol. 2024 Sep 20. doi: 10.1007/s42770-024-01518-4. Online ahead of print. Braz J Microbiol. 2024. PMID: 39302629
-
Variant-specific antibody profiling for tracking SARS-CoV-2 variant infections in children and adolescents.Front Immunol. 2024 Aug 27;15:1434291. doi: 10.3389/fimmu.2024.1434291. eCollection 2024. Front Immunol. 2024. PMID: 39257574 Free PMC article.
-
Molecular basis of convergent evolution of ACE2 receptor utilization among HKU5 coronaviruses.bioRxiv [Preprint]. 2024 Aug 28:2024.08.28.608351. doi: 10.1101/2024.08.28.608351. bioRxiv. 2024. PMID: 39253417 Free PMC article. Preprint.
-
Passive infusion of an S2-Stem broadly neutralizing antibody protects against SARS-CoV-2 infection and lower airway inflammation in rhesus macaques.bioRxiv [Preprint]. 2024 Jul 30:2024.07.30.605768. doi: 10.1101/2024.07.30.605768. bioRxiv. 2024. PMID: 39109178 Free PMC article. Preprint.
References
Publication types
MeSH terms
Substances
Grants and funding
LinkOut - more resources
Full Text Sources
Other Literature Sources
Medical
Miscellaneous