Microfluidic platform accelerates tissue processing into single cells for molecular analysis and primary culture models
- PMID: 34001902
- PMCID: PMC8128882
- DOI: 10.1038/s41467-021-23238-1
Microfluidic platform accelerates tissue processing into single cells for molecular analysis and primary culture models
Abstract
Tissues are complex mixtures of different cell subtypes, and this diversity is increasingly characterized using high-throughput single cell analysis methods. However, these efforts are hindered, as tissues must first be dissociated into single cell suspensions using methods that are often inefficient, labor-intensive, highly variable, and potentially biased towards certain cell subtypes. Here, we present a microfluidic platform consisting of three tissue processing technologies that combine tissue digestion, disaggregation, and filtration. The platform is evaluated using a diverse array of tissues. For kidney and mammary tumor, microfluidic processing produces 2.5-fold more single cells. Single cell RNA sequencing further reveals that endothelial cells, fibroblasts, and basal epithelium are enriched without affecting stress response. For liver and heart, processing time is dramatically reduced. We also demonstrate that recovery of cells from the system at periodic intervals during processing increases hepatocyte and cardiomyocyte numbers, as well as increases reproducibility from batch-to-batch for all tissues.
Conflict of interest statement
J. B. H. is a co-founder of Kino Discovery, which is in the process of licensing intellectual property for the tissue processing devices. The other authors declare no competing interests.
Figures
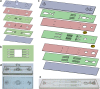
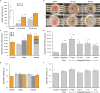
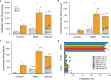
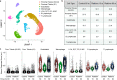
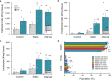
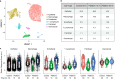
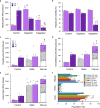
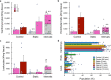
Similar articles
-
Transcriptional and Cellular Diversity of the Human Heart.Circulation. 2020 Aug 4;142(5):466-482. doi: 10.1161/CIRCULATIONAHA.119.045401. Epub 2020 May 14. Circulation. 2020. PMID: 32403949 Free PMC article.
-
HeLiVa platform: integrated heart-liver-vascular systems for drug testing in human health and disease.Stem Cell Res Ther. 2013;4 Suppl 1(Suppl 1):S8. doi: 10.1186/scrt369. Epub 2013 Dec 20. Stem Cell Res Ther. 2013. PMID: 24565063 Free PMC article. Review.
-
Microfluidic filter device with nylon mesh membranes efficiently dissociates cell aggregates and digested tissue into single cells.Lab Chip. 2018 Sep 11;18(18):2776-2786. doi: 10.1039/c8lc00507a. Lab Chip. 2018. PMID: 30090895 Free PMC article.
-
Single-Cell Sequencing of the Healthy and Diseased Heart Reveals Cytoskeleton-Associated Protein 4 as a New Modulator of Fibroblasts Activation.Circulation. 2018 Jul 10;138(2):166-180. doi: 10.1161/CIRCULATIONAHA.117.030742. Epub 2018 Jan 31. Circulation. 2018. PMID: 29386203
-
Microfluidic platforms for hepatocyte cell culture: new technologies and applications.Ann Biomed Eng. 2012 Jun;40(6):1244-54. doi: 10.1007/s10439-011-0453-8. Epub 2011 Oct 29. Ann Biomed Eng. 2012. PMID: 22042626 Review.
Cited by
-
Microfluidic manipulation by spiral hollow-fibre actuators.Nat Commun. 2022 Mar 14;13(1):1331. doi: 10.1038/s41467-022-29088-9. Nat Commun. 2022. PMID: 35288561 Free PMC article.
-
Drosophila CASK regulates brain size and neuronal morphogenesis, providing a genetic model of postnatal microcephaly suitable for drug discovery.Neural Dev. 2023 Oct 7;18(1):6. doi: 10.1186/s13064-023-00174-y. Neural Dev. 2023. PMID: 37805506 Free PMC article.
-
Single-cell RNA sequencing in diabetic kidney disease: a literature review.Ren Fail. 2024 Dec;46(2):2387428. doi: 10.1080/0886022X.2024.2387428. Epub 2024 Aug 4. Ren Fail. 2024. PMID: 39099183 Free PMC article. Review.
-
Best Practices and Progress in Precision-Cut Liver Slice Cultures.Int J Mol Sci. 2021 Jul 1;22(13):7137. doi: 10.3390/ijms22137137. Int J Mol Sci. 2021. PMID: 34281187 Free PMC article. Review.
-
Magnetophoretic circuits: A review of device designs and implementation for precise single-cell manipulation.Anal Chim Acta. 2023 Sep 1;1272:341425. doi: 10.1016/j.aca.2023.341425. Epub 2023 May 31. Anal Chim Acta. 2023. PMID: 37355317 Free PMC article. Review.
References
Publication types
MeSH terms
Grants and funding
LinkOut - more resources
Full Text Sources
Other Literature Sources
Molecular Biology Databases