Impaired ATG16L-Dependent Autophagy Promotes Renal Interstitial Fibrosis in Chronic Renal Graft Dysfunction Through Inducing EndMT by NF-κB Signal Pathway
- PMID: 33927720
- PMCID: PMC8076642
- DOI: 10.3389/fimmu.2021.650424
Impaired ATG16L-Dependent Autophagy Promotes Renal Interstitial Fibrosis in Chronic Renal Graft Dysfunction Through Inducing EndMT by NF-κB Signal Pathway
Abstract
Chronic renal graft dysfunction (CAD) is caused by multiple factors, including glomerular sclerosis, inflammation, interstitial fibrosis and tubular atrophy (IF/TA). However, the most prominent elements of CAD are IF/TA. Our studies have confirmed that endothelial-mesenchymal transition (EndMT) is an important source to allograft IF/TA. The characteristic of EndMT is the loss of endothelial marker and the acquisition of mesenchymal or fibroblastic phenotypes. Autophagy is an intracellular degradation pathway that is regulated by autophagy-related proteins and plays a vital role in many fibrotic conditions. However, whether or not autophagy contributes to fibrosis of renal allograft and how such mechanism occurs still remains unclear. Autophagy related 16 like gene (ATG16L) is a critical autophagy-related gene (ARG) necessary for autophagosome formation. Here, we first analyzed kidney transplant patient tissues from Gene Expression Omnibus (GEO) datasets and 60 transplant patients from our center. Recipients with stable kidney function were defined as non-CAD group and all patients in CAD group were histopathologically diagnosed with CAD. Results showed that ATG16L, as one significant differential ARG, was less expressed in CAD group compared to the non-CAD group. Furthermore, we found there were less autophagosomes and autolysosomes in transplanted kidneys of CAD patients, and downregulation of autophagy is a poor prognostic factor. In vitro, we found out that the knockdown of ATG16L enhanced the process of EndMT in human renal glomerular endothelial cells (HRGECs). In vivo, the changes of EndMT and autophagic flux were then detected in rat renal transplant models of CAD. We demonstrated the occurrence of EndMT, and indicated that abundance of ATG16L was accompanied by the dynamic autophagic flux change along different stages of kidney transplantation. Mechanistically, knockdown of ATG16L, specifically in endothelial cells, reduced of NF-κB degradation and excreted inflammatory cytokines (IL-1β, IL-6 and TNF-α), which could facilitate EndMT. In conclusion, ATG16L-dependent autophagic flux causing by transplant showed progressive loss increase over time. Inflammatory cytokines from this process promoted EndMT, thereby leading to progression of CAD. ATG16L served as a negative regulator of EndMT and development of renal graft fibrosis, and autophagy can be explored as a potential therapeutic target for chronic renal graft dysfunction.
Keywords: ATG16L; EndMT; autophagy; chronic renal graft dysfunction; inflammatory cytokines; renal interstitial fibrosis.
Copyright © 2021 Gui, Suo, Wang, Zheng, Fei, Chen, Sun, Han, Tao, Ju, Yang, Gu and Tan.
Conflict of interest statement
The authors declare that the research was conducted in the absence of any commercial or financial relationships that could be construed as a potential conflict of interest.
Figures
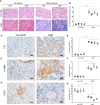
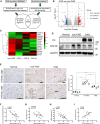
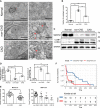

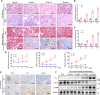
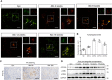
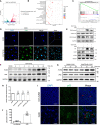
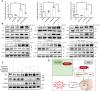
Similar articles
-
Everolimus Alleviates Renal Allograft Interstitial Fibrosis by Inhibiting Epithelial-to-Mesenchymal Transition Not Only via Inducing Autophagy but Also via Stabilizing IκB-α.Front Immunol. 2022 Jan 24;12:753412. doi: 10.3389/fimmu.2021.753412. eCollection 2021. Front Immunol. 2022. PMID: 35140705 Free PMC article.
-
Curcumin Blunts IL-6 Dependent Endothelial-to-Mesenchymal Transition to Alleviate Renal Allograft Fibrosis Through Autophagy Activation.Front Immunol. 2021 May 28;12:656242. doi: 10.3389/fimmu.2021.656242. eCollection 2021. Front Immunol. 2021. Retraction in: Front Immunol. 2021 Nov 26;12:818539. doi: 10.3389/fimmu.2021.818539 PMID: 34122411 Free PMC article. Retracted.
-
Rictor/mTORC2 signalling contributes to renal vascular endothelial-to-mesenchymal transition and renal allograft interstitial fibrosis by regulating BNIP3-mediated mitophagy.Clin Transl Med. 2024 May;14(5):e1686. doi: 10.1002/ctm2.1686. Clin Transl Med. 2024. PMID: 38769658 Free PMC article.
-
Endothelial-to-mesenchymal transition: Cytokine-mediated pathways that determine endothelial fibrosis under inflammatory conditions.Cytokine Growth Factor Rev. 2017 Feb;33:41-54. doi: 10.1016/j.cytogfr.2016.09.002. Epub 2016 Sep 23. Cytokine Growth Factor Rev. 2017. PMID: 27692608 Review.
-
Role of Endothelial Cells in Renal Fibrosis.Adv Exp Med Biol. 2019;1165:145-163. doi: 10.1007/978-981-13-8871-2_8. Adv Exp Med Biol. 2019. PMID: 31399965 Review.
Cited by
-
Construction of predictive model of interstitial fibrosis and tubular atrophy after kidney transplantation with machine learning algorithms.Front Genet. 2023 Nov 1;14:1276963. doi: 10.3389/fgene.2023.1276963. eCollection 2023. Front Genet. 2023. PMID: 38028591 Free PMC article.
-
Macrophage polarization induces endothelium-to-myofibroblast transition in chronic allograft dysfunction.Ren Fail. 2023 Dec;45(1):2220418. doi: 10.1080/0886022X.2023.2220418. Ren Fail. 2023. PMID: 37288756 Free PMC article.
-
The nuclear factor kappa B signaling pathway is a master regulator of renal fibrosis.Front Pharmacol. 2024 Jan 16;14:1335094. doi: 10.3389/fphar.2023.1335094. eCollection 2023. Front Pharmacol. 2024. PMID: 38293668 Free PMC article. Review.
-
Inhibitory Effects of Rhein on Renal Interstitial Fibrosis via the SHH-Gli1 Signal Pathway.Evid Based Complement Alternat Med. 2022 Aug 5;2022:4398265. doi: 10.1155/2022/4398265. eCollection 2022. Evid Based Complement Alternat Med. 2022. PMID: 35966731 Free PMC article.
-
Amelioration of Renal Injury by Resveratrol in a Rat Renal Transplantation Model via Activation of the SIRT1/NF-κB Signaling Pathway.Biomed Res Int. 2022 Mar 28;2022:7140961. doi: 10.1155/2022/7140961. eCollection 2022. Biomed Res Int. 2022. PMID: 35386302 Free PMC article.
References
Publication types
MeSH terms
Substances
LinkOut - more resources
Full Text Sources
Other Literature Sources
Medical
Miscellaneous