VPS13D bridges the ER to mitochondria and peroxisomes via Miro
- PMID: 33891013
- PMCID: PMC8077184
- DOI: 10.1083/jcb.202010004
VPS13D bridges the ER to mitochondria and peroxisomes via Miro
Erratum in
-
Correction: VPS13D bridges the ER to mitochondria and peroxisomes via Miro.J Cell Biol. 2021 Aug 2;220(8):e20201000405052021c. doi: 10.1083/jcb.20201000405052021c. Epub 2021 Jun 22. J Cell Biol. 2021. PMID: 34156432 Free PMC article. No abstract available.
Abstract
Mitochondria, which are excluded from the secretory pathway, depend on lipid transport proteins for their lipid supply from the ER, where most lipids are synthesized. In yeast, the outer mitochondrial membrane GTPase Gem1 is an accessory factor of ERMES, an ER-mitochondria tethering complex that contains lipid transport domains and that functions, partially redundantly with Vps13, in lipid transfer between the two organelles. In metazoa, where VPS13, but not ERMES, is present, the Gem1 orthologue Miro was linked to mitochondrial dynamics but not to lipid transport. Here we show that Miro, including its peroxisome-enriched splice variant, recruits the lipid transport protein VPS13D, which in turn binds the ER in a VAP-dependent way and thus could provide a lipid conduit between the ER and mitochondria. These findings reveal a so far missing link between function(s) of Gem1/Miro in yeast and higher eukaryotes, where Miro is a Parkin substrate, with potential implications for Parkinson's disease pathogenesis.
© 2021 Guillén-Samander et al.
Figures
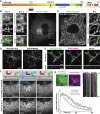
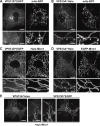
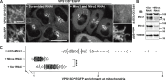
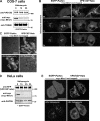
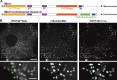
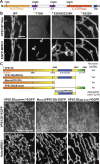
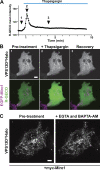
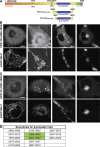
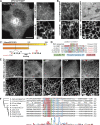
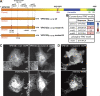
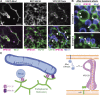
Similar articles
-
The conserved GTPase Gem1 regulates endoplasmic reticulum-mitochondria connections.Proc Natl Acad Sci U S A. 2011 Aug 23;108(34):14151-6. doi: 10.1073/pnas.1111314108. Epub 2011 Aug 8. Proc Natl Acad Sci U S A. 2011. PMID: 21825164 Free PMC article.
-
The ERMES complex and ER-mitochondria connections.Biochem Soc Trans. 2012 Apr;40(2):445-50. doi: 10.1042/BST20110758. Biochem Soc Trans. 2012. PMID: 22435828 Review.
-
VPS13D interacts with VCP/p97 and negatively regulates endoplasmic reticulum-mitochondria interactions.Mol Biol Cell. 2021 Aug 1;32(16):1474-1486. doi: 10.1091/mbc.E21-03-0097. Epub 2021 Jun 16. Mol Biol Cell. 2021. PMID: 34133214 Free PMC article.
-
Gem1 and ERMES do not directly affect phosphatidylserine transport from ER to mitochondria or mitochondrial inheritance.Traffic. 2012 Jun;13(6):880-90. doi: 10.1111/j.1600-0854.2012.01352.x. Epub 2012 Apr 8. Traffic. 2012. PMID: 22409400 Free PMC article.
-
Mitochondrial Miro GTPases coordinate mitochondrial and peroxisomal dynamics.Small GTPases. 2021 Sep-Nov;12(5-6):372-398. doi: 10.1080/21541248.2020.1843957. Epub 2020 Nov 12. Small GTPases. 2021. PMID: 33183150 Free PMC article. Review.
Cited by
-
Shared structural features of Miro binding control mitochondrial homeostasis.EMBO J. 2024 Feb;43(4):595-614. doi: 10.1038/s44318-024-00028-1. Epub 2024 Jan 24. EMBO J. 2024. PMID: 38267654 Free PMC article.
-
ESCRT dysfunction compromises endoplasmic reticulum maturation and autophagosome biogenesis in Drosophila.Curr Biol. 2022 Mar 28;32(6):1262-1274.e4. doi: 10.1016/j.cub.2022.01.040. Epub 2022 Feb 7. Curr Biol. 2022. PMID: 35134326 Free PMC article.
-
VPS13D promotes peroxisome biogenesis.J Cell Biol. 2021 May 3;220(5):e202001188. doi: 10.1083/jcb.202001188. J Cell Biol. 2021. PMID: 33891012 Free PMC article.
-
The peroxisome: an update on mysteries 3.0.Histochem Cell Biol. 2024 Feb;161(2):99-132. doi: 10.1007/s00418-023-02259-5. Epub 2024 Jan 20. Histochem Cell Biol. 2024. PMID: 38244103 Free PMC article. Review.
-
VPS13B is localized at the cis-trans Golgi complex interface and is a functional partner of FAM177A1.bioRxiv [Preprint]. 2023 Dec 18:2023.12.18.572081. doi: 10.1101/2023.12.18.572081. bioRxiv. 2023. Update in: J Cell Biol. 2024 Dec 2;223(12):e202311189. doi: 10.1083/jcb.202311189 PMID: 38187698 Free PMC article. Updated. Preprint.
References
-
- Akşit, A. 2018. Peroxisomal Membrane Contact Sites in the Yeast Hansenula Polymorpha. PhD Thesis. University of Groningen, Groningen, Netherlands. Available at: https://research.rug.nl/en/publications/peroxisomal-membrane-contact-sit....
-
- Anding, A.L., Wang C., Chang T.-K., Sliter D.A., Powers C.M., Hofmann K., Youle R.J., and Baehrecke E.H.. 2018. Vps13D encodes a ubiquitin-binding protein that is required for the regulation of mitochondrial size and clearance. Curr. Biol. 28:287–295.e6. 10.1016/j.cub.2017.11.064 - DOI - PMC - PubMed
Publication types
MeSH terms
Substances
Grants and funding
LinkOut - more resources
Full Text Sources
Other Literature Sources
Molecular Biology Databases
Research Materials