On the molecular nature of large-pore channels
- PMID: 33865869
- PMCID: PMC8409005
- DOI: 10.1016/j.jmb.2021.166994
On the molecular nature of large-pore channels
Abstract
Membrane transport is a fundamental means to control basic cellular processes such as apoptosis, inflammation, and neurodegeneration and is mediated by a number of transporters, pumps, and channels. Accumulating evidence over the last half century has shown that a type of so-called "large-pore channel" exists in various tissues and organs in gap-junctional and non-gap-junctional forms in order to flow not only ions but also metabolites such as ATP. They are formed by a number of protein families with little or no evolutionary linkages including connexin, innexin, pannexin, leucine-rich repeat-containing 8 (LRRC8), and calcium homeostasis modulator (CALHM). This review summarizes the history and concept of large-pore channels starting from connexin gap junction channels to the more recent developments in innexin, pannexin, LRRC8, and CALHM. We describe structural and functional features of large-pore channels that are crucial for their diverse functions on the basis of available structures.
Keywords: CALHM; Connexin; Innexin; LRRC8; Pannexin.
Copyright © 2021 Elsevier Ltd. All rights reserved.
Conflict of interest statement
Declaration of interests
The authors declare no competing interests.
Figures
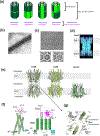
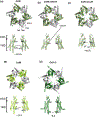
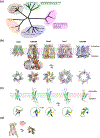
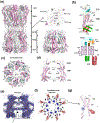
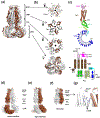
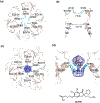
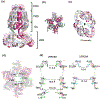
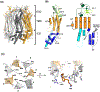
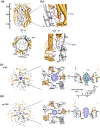
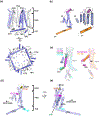
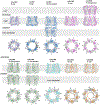
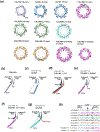
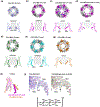
Similar articles
-
Contribution of large-pore channels to inflammation induced by microorganisms.Front Cell Dev Biol. 2023 Jan 9;10:1094362. doi: 10.3389/fcell.2022.1094362. eCollection 2022. Front Cell Dev Biol. 2023. PMID: 36699007 Free PMC article. Review.
-
Calcium homeostasis modulator (CALHM) ion channels.Pflugers Arch. 2016 Mar;468(3):395-403. doi: 10.1007/s00424-015-1757-6. Epub 2015 Nov 25. Pflugers Arch. 2016. PMID: 26603282 Free PMC article. Review.
-
Pannexin channels are not gap junction hemichannels.Channels (Austin). 2011 May-Jun;5(3):193-7. doi: 10.4161/chan.5.3.15765. Epub 2011 May 1. Channels (Austin). 2011. PMID: 21532340 Free PMC article. Review.
-
Connexin and Pannexin Large-Pore Channels in Microcirculation and Neurovascular Coupling Function.Int J Mol Sci. 2022 Jun 30;23(13):7303. doi: 10.3390/ijms23137303. Int J Mol Sci. 2022. PMID: 35806312 Free PMC article. Review.
-
Emerging issues of connexin channels: biophysics fills the gap.Q Rev Biophys. 2001 Aug;34(3):325-472. doi: 10.1017/s0033583501003705. Q Rev Biophys. 2001. PMID: 11838236 Review.
Cited by
-
The ATP-exporting channel Pannexin-1 promotes CD8+ T cell effector and memory responses.bioRxiv [Preprint]. 2024 May 2:2023.04.19.537580. doi: 10.1101/2023.04.19.537580. bioRxiv. 2024. Update in: iScience. 2024 Jun 17;27(7):110290. doi: 10.1016/j.isci.2024.110290 PMID: 37131831 Free PMC article. Updated. Preprint.
-
Mefloquine-induced conformational shift in Cx36 N-terminal helix leading to channel closure mediated by lipid bilayer.Nat Commun. 2024 Oct 25;15(1):9223. doi: 10.1038/s41467-024-53587-6. Nat Commun. 2024. PMID: 39455592 Free PMC article.
-
Contribution of large-pore channels to inflammation induced by microorganisms.Front Cell Dev Biol. 2023 Jan 9;10:1094362. doi: 10.3389/fcell.2022.1094362. eCollection 2022. Front Cell Dev Biol. 2023. PMID: 36699007 Free PMC article. Review.
-
ATP-releasing SWELL1 channel in spinal microglia contributes to neuropathic pain.Sci Adv. 2023 Mar 29;9(13):eade9931. doi: 10.1126/sciadv.ade9931. Epub 2023 Mar 29. Sci Adv. 2023. PMID: 36989353 Free PMC article.
-
Connexin Gap Junction Channels and Hemichannels: Insights from High-Resolution Structures.Biology (Basel). 2024 Apr 26;13(5):298. doi: 10.3390/biology13050298. Biology (Basel). 2024. PMID: 38785780 Free PMC article. Review.
References
-
- Bevans CG, Kordel M, Rhee SK, Harris AL. Isoform composition of connexin channels determines selectivity among second messengers and uncharged molecules. J Biol Chem. 1998;273:2808–16. - PubMed
-
- Goldberg GS, Lampe PD, Nicholson BJ. Selective transfer of endogenous metabolites through gap junctions composed of different connexins. Nat Cell Biol. 1999;1:457–9. - PubMed
-
- Goodenough DA, Paul DL. Gap Junctions. 2019.
Publication types
MeSH terms
Substances
Grants and funding
LinkOut - more resources
Full Text Sources
Other Literature Sources
Miscellaneous