Missense mutation of Fmr1 results in impaired AMPAR-mediated plasticity and socio-cognitive deficits in mice
- PMID: 33692361
- PMCID: PMC7946954
- DOI: 10.1038/s41467-021-21820-1
Missense mutation of Fmr1 results in impaired AMPAR-mediated plasticity and socio-cognitive deficits in mice
Abstract
Fragile X syndrome (FXS) is the most frequent form of inherited intellectual disability and the best-described monogenic cause of autism. CGG-repeat expansion in the FMR1 gene leads to FMR1 silencing, loss-of-expression of the Fragile X Mental Retardation Protein (FMRP), and is a common cause of FXS. Missense mutations in the FMR1 gene were also identified in FXS patients, including the recurrent FMRP-R138Q mutation. To investigate the mechanisms underlying FXS caused by this mutation, we generated a knock-in mouse model (Fmr1R138Q) expressing the FMRP-R138Q protein. We demonstrate that, in the hippocampus of the Fmr1R138Q mice, neurons show an increased spine density associated with synaptic ultrastructural defects and increased AMPA receptor-surface expression. Combining biochemical assays, high-resolution imaging, electrophysiological recordings, and behavioural testing, we also show that the R138Q mutation results in impaired hippocampal long-term potentiation and socio-cognitive deficits in mice. These findings reveal the functional impact of the FMRP-R138Q mutation in a mouse model of FXS.
Conflict of interest statement
The authors declare no competing interests.
Figures
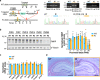
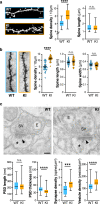
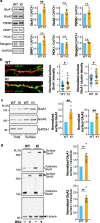
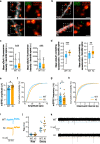
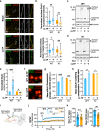
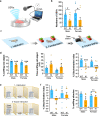
Similar articles
-
Selective Deletion of Astroglial FMRP Dysregulates Glutamate Transporter GLT1 and Contributes to Fragile X Syndrome Phenotypes In Vivo.J Neurosci. 2016 Jul 6;36(27):7079-94. doi: 10.1523/JNEUROSCI.1069-16.2016. J Neurosci. 2016. PMID: 27383586 Free PMC article.
-
Independent role for presynaptic FMRP revealed by an FMR1 missense mutation associated with intellectual disability and seizures.Proc Natl Acad Sci U S A. 2015 Jan 27;112(4):949-56. doi: 10.1073/pnas.1423094112. Epub 2015 Jan 5. Proc Natl Acad Sci U S A. 2015. PMID: 25561520 Free PMC article.
-
Epigenetic characterization of the FMR1 gene and aberrant neurodevelopment in human induced pluripotent stem cell models of fragile X syndrome.PLoS One. 2011;6(10):e26203. doi: 10.1371/journal.pone.0026203. Epub 2011 Oct 12. PLoS One. 2011. PMID: 22022567 Free PMC article.
-
Hippocampal dysfunction and cognitive impairment in Fragile-X Syndrome.Neurosci Biobehav Rev. 2016 Sep;68:563-574. doi: 10.1016/j.neubiorev.2016.06.033. Epub 2016 Jun 23. Neurosci Biobehav Rev. 2016. PMID: 27345143 Review.
-
Rare FMR1 gene mutations causing fragile X syndrome: A review.Am J Med Genet A. 2018 Jan;176(1):11-18. doi: 10.1002/ajmg.a.38504. Epub 2017 Nov 27. Am J Med Genet A. 2018. PMID: 29178241 Free PMC article. Review.
Cited by
-
RNF220 is an E3 ubiquitin ligase for AMPA receptors to regulate synaptic transmission.Sci Adv. 2022 Sep 30;8(39):eabq4736. doi: 10.1126/sciadv.abq4736. Epub 2022 Sep 30. Sci Adv. 2022. PMID: 36179027 Free PMC article.
-
New Animal Models for Understanding FMRP Functions and FXS Pathology.Cells. 2022 May 12;11(10):1628. doi: 10.3390/cells11101628. Cells. 2022. PMID: 35626665 Free PMC article. Review.
-
FMRP Sustains Presynaptic Function via Control of Activity-Dependent Bulk Endocytosis.J Neurosci. 2022 Feb 23;42(8):1618-1628. doi: 10.1523/JNEUROSCI.0852-21.2021. Epub 2022 Jan 7. J Neurosci. 2022. PMID: 34996816 Free PMC article.
-
Case report: genetic analysis of a novel frameshift mutation in FMR1 gene in a Chinese family.Front Genet. 2023 Sep 7;14:1228682. doi: 10.3389/fgene.2023.1228682. eCollection 2023. Front Genet. 2023. PMID: 37745859 Free PMC article.
-
Investigation of Interferon Gamma Activity Using Bioinformatics Methods.Arch Razi Inst. 2021 Nov 30;76(5):1245-1253. doi: 10.22092/ari.2021.356106.1780. eCollection 2021 Nov. Arch Razi Inst. 2021. PMID: 35355749 Free PMC article.
References
Publication types
MeSH terms
Substances
LinkOut - more resources
Full Text Sources
Other Literature Sources
Molecular Biology Databases