VEGFA165 gene therapy ameliorates blood-labyrinth barrier breakdown and hearing loss
- PMID: 33690221
- PMCID: PMC8119217
- DOI: 10.1172/jci.insight.143285
VEGFA165 gene therapy ameliorates blood-labyrinth barrier breakdown and hearing loss
Abstract
Millions of people are affected by hearing loss. Hearing loss is frequently caused by noise or aging and often associated with loss of pericytes. Pericytes populate the small vessels in the adult cochlea. However, their role in different types of hearing loss is largely unknown. Using an inducible and conditional pericyte depletion mouse model and noise-exposed mouse model, we show that loss of pericytes leads to marked changes in vascular structure, in turn leading to vascular degeneration and hearing loss. In vitro, using advanced tissue explants from pericyte fluorescence reporter models combined with exogenous donor pericytes, we show that pericytes, signaled by VEGF isoform A165 (VEGFA165), vigorously drive new vessel growth in both adult and neonatal mouse inner ear tissue. In vivo, the delivery of an adeno-associated virus serotype 1-mediated (AAV1-mediated) VEGFA165 viral vector to pericyte-depleted or noise-exposed animals prevented and regenerated lost pericytes, improved blood supply, and attenuated hearing loss. These studies provide the first clear-cut evidence that pericytes are critical for vascular regeneration, vascular stability, and hearing in adults. The restoration of vascular function in the damaged cochlea, including in noise-exposed animals, suggests that VEGFA165 gene therapy could be a new strategy for ameliorating vascular associated hearing disorders.
Keywords: Angiogenesis; Cardiovascular disease.
Conflict of interest statement
Figures
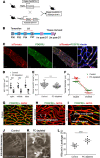
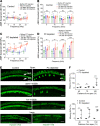
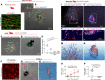
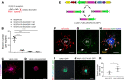
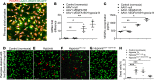
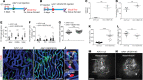
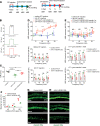
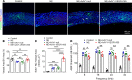
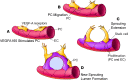
Similar articles
-
Vascular regeneration in adult mouse cochlea stimulated by VEGF-A165 and driven by NG2-derived cells ex vivo.Hear Res. 2019 Jun;377:179-188. doi: 10.1016/j.heares.2019.03.010. Epub 2019 Mar 29. Hear Res. 2019. PMID: 30954884 Free PMC article.
-
Cochlear pericyte responses to acoustic trauma and the involvement of hypoxia-inducible factor-1alpha and vascular endothelial growth factor.Am J Pathol. 2009 May;174(5):1692-704. doi: 10.2353/ajpath.2009.080739. Epub 2009 Apr 6. Am J Pathol. 2009. PMID: 19349367 Free PMC article.
-
Acoustic Trauma Causes Cochlear Pericyte-to-Myofibroblast-Like Cell Transformation and Vascular Degeneration, and Transplantation of New Pericytes Prevents Vascular Atrophy.Am J Pathol. 2020 Sep;190(9):1943-1959. doi: 10.1016/j.ajpath.2020.05.019. Epub 2020 Jun 18. Am J Pathol. 2020. PMID: 32562655 Free PMC article.
-
Pathophysiology of the cochlear intrastrial fluid-blood barrier (review).Hear Res. 2016 Aug;338:52-63. doi: 10.1016/j.heares.2016.01.010. Epub 2016 Jan 20. Hear Res. 2016. PMID: 26802581 Free PMC article. Review.
-
Research advances in cochlear pericytes and hearing loss.Hear Res. 2023 Oct;438:108877. doi: 10.1016/j.heares.2023.108877. Epub 2023 Aug 19. Hear Res. 2023. PMID: 37651921 Free PMC article. Review.
Cited by
-
Nucleic Acid Delivery to the Vascular Endothelium.Mol Pharm. 2022 Dec 5;19(12):4466-4486. doi: 10.1021/acs.molpharmaceut.2c00653. Epub 2022 Oct 17. Mol Pharm. 2022. PMID: 36251765 Free PMC article. Review.
-
Protein isoform-centric therapeutics: expanding targets and increasing specificity.Nat Rev Drug Discov. 2024 Oct;23(10):759-779. doi: 10.1038/s41573-024-01025-z. Epub 2024 Sep 4. Nat Rev Drug Discov. 2024. PMID: 39232238 Review.
-
The role of the stria vascularis in neglected otologic disease.Hear Res. 2023 Feb;428:108682. doi: 10.1016/j.heares.2022.108682. Epub 2022 Dec 24. Hear Res. 2023. PMID: 36584545 Free PMC article. Review.
-
Innate Immune Response to Viral Vectors in Gene Therapy.Viruses. 2023 Aug 24;15(9):1801. doi: 10.3390/v15091801. Viruses. 2023. PMID: 37766208 Free PMC article. Review.
-
Stretch regulates alveologenesis and homeostasis via mesenchymal Gαq/11-mediated TGFβ2 activation.Development. 2023 May 1;150(9):dev201046. doi: 10.1242/dev.201046. Epub 2023 May 12. Development. 2023. PMID: 37102682 Free PMC article.
References
-
- Neng L, Shi X. Vascular pathology and hearing disorders. Curr Opin Physiol. 2020;18:79–84. doi: 10.1016/j.cophys.2020.09.004. - DOI
Publication types
MeSH terms
Substances
Grants and funding
LinkOut - more resources
Full Text Sources
Other Literature Sources
Molecular Biology Databases
Research Materials