Epstein-Barr virus inactivates the transcriptome and disrupts the chromatin architecture of its host cell in the first phase of lytic reactivation
- PMID: 33675667
- PMCID: PMC8034645
- DOI: 10.1093/nar/gkab099
Epstein-Barr virus inactivates the transcriptome and disrupts the chromatin architecture of its host cell in the first phase of lytic reactivation
Abstract
Epstein-Barr virus (EBV), a herpes virus also termed HHV 4 and the first identified human tumor virus, establishes a stable, long-term latent infection in human B cells, its preferred host. Upon induction of EBV's lytic phase, the latently infected cells turn into a virus factory, a process that is governed by EBV. In the lytic, productive phase, all herpes viruses ensure the efficient induction of all lytic viral genes to produce progeny, but certain of these genes also repress the ensuing antiviral responses of the virally infected host cells, regulate their apoptotic death or control the cellular transcriptome. We now find that EBV causes previously unknown massive and global alterations in the chromatin of its host cell upon induction of the viral lytic phase and prior to the onset of viral DNA replication. The viral initiator protein of the lytic cycle, BZLF1, binds to >105 binding sites with different sequence motifs in cellular chromatin in a concentration dependent manner implementing a binary molar switch probably to prevent noise-induced erroneous induction of EBV's lytic phase. Concomitant with DNA binding of BZLF1, silent chromatin opens locally as shown by ATAC-seq experiments, while previously wide-open cellular chromatin becomes inaccessible on a global scale within hours. While viral transcripts increase drastically, the induction of the lytic phase results in a massive reduction of cellular transcripts and a loss of chromatin-chromatin interactions of cellular promoters with their distal regulatory elements as shown in Capture-C experiments. Our data document that EBV's lytic cycle induces discrete early processes that disrupt the architecture of host cellular chromatin and repress the cellular epigenome and transcriptome likely supporting the efficient de novo synthesis of this herpes virus.
© The Author(s) 2021. Published by Oxford University Press on behalf of Nucleic Acids Research.
Figures
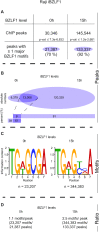
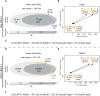
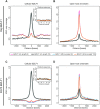
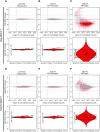
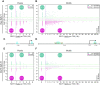
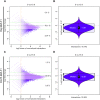
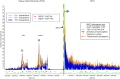
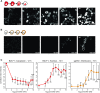
Similar articles
-
How Epstein-Barr Virus Induces the Reorganization of Cellular Chromatin.mBio. 2023 Feb 28;14(1):e0268622. doi: 10.1128/mbio.02686-22. Epub 2023 Jan 10. mBio. 2023. PMID: 36625581 Free PMC article.
-
The lytic phase of epstein-barr virus requires a viral genome with 5-methylcytosine residues in CpG sites.J Virol. 2012 Jan;86(1):447-58. doi: 10.1128/JVI.06314-11. Epub 2011 Oct 26. J Virol. 2012. PMID: 22031942 Free PMC article.
-
BZLF1 governs CpG-methylated chromatin of Epstein-Barr Virus reversing epigenetic repression.PLoS Pathog. 2012 Sep;8(9):e1002902. doi: 10.1371/journal.ppat.1002902. Epub 2012 Sep 6. PLoS Pathog. 2012. PMID: 22969425 Free PMC article.
-
Epigenetic lifestyle of Epstein-Barr virus.Semin Immunopathol. 2020 Apr;42(2):131-142. doi: 10.1007/s00281-020-00792-2. Epub 2020 Mar 30. Semin Immunopathol. 2020. PMID: 32232535 Free PMC article. Review.
-
Latent and lytic Epstein-Barr virus replication strategies.Rev Med Virol. 2005 Jan-Feb;15(1):3-15. doi: 10.1002/rmv.441. Rev Med Virol. 2005. PMID: 15386591 Review.
Cited by
-
How Epstein-Barr Virus Induces the Reorganization of Cellular Chromatin.mBio. 2023 Feb 28;14(1):e0268622. doi: 10.1128/mbio.02686-22. Epub 2023 Jan 10. mBio. 2023. PMID: 36625581 Free PMC article.
-
Epigenetic control of the Epstein-Barr lifecycle.Curr Opin Virol. 2022 Feb;52:78-88. doi: 10.1016/j.coviro.2021.11.013. Epub 2021 Dec 8. Curr Opin Virol. 2022. PMID: 34891084 Free PMC article. Review.
-
Epstein-Barr virus induces host shutoff extensively via BGLF5-independent mechanisms.Cell Rep. 2024 Oct 22;43(10):114743. doi: 10.1016/j.celrep.2024.114743. Epub 2024 Sep 18. Cell Rep. 2024. PMID: 39298313 Free PMC article.
-
SARS-CoV-2 and Epstein-Barr Virus-like Particles Associate and Fuse with Extracellular Vesicles in Virus Neutralization Tests.Biomedicines. 2023 Oct 25;11(11):2892. doi: 10.3390/biomedicines11112892. Biomedicines. 2023. PMID: 38001893 Free PMC article.
-
EBV Reactivation from Latency Is a Degrading Experience for the Host.Viruses. 2023 Mar 11;15(3):726. doi: 10.3390/v15030726. Viruses. 2023. PMID: 36992435 Free PMC article. Review.
References
-
- Quinlan M.P., Chen L.B., Knipe D.M.. The intranuclear location of a herpes simplex virus DNA-binding protein is determined by the status of viral DNA replication. Cell. 1984; 36:857–868. - PubMed
Publication types
MeSH terms
Substances
Grants and funding
LinkOut - more resources
Full Text Sources
Other Literature Sources