Mechanisms of nonvesicular lipid transport
- PMID: 33605998
- PMCID: PMC7901144
- DOI: 10.1083/jcb.202012058
Mechanisms of nonvesicular lipid transport
Abstract
We have long known that lipids traffic between cellular membranes via vesicles but have only recently appreciated the role of nonvesicular lipid transport. Nonvesicular transport can be high volume, supporting biogenesis of rapidly expanding membranes, or more targeted and precise, allowing cells to rapidly alter levels of specific lipids in membranes. Most such transport probably occurs at membrane contact sites, where organelles are closely apposed, and requires lipid transport proteins (LTPs), which solubilize lipids to shield them from the aqueous phase during their transport between membranes. Some LTPs are cup like and shuttle lipid monomers between membranes. Others form conduits allowing lipid flow between membranes. This review describes what we know about nonvesicular lipid transfer mechanisms while also identifying many remaining unknowns: How do LTPs facilitate lipid movement from and into membranes, do LTPs require accessory proteins for efficient transfer in vivo, and how is directionality of transport determined?
© 2021 Reinisch and Prinz.
Figures
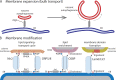
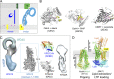
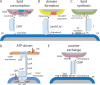
Similar articles
-
Nonvesicular lipid transfer from the endoplasmic reticulum.Cold Spring Harb Perspect Biol. 2012 Oct 1;4(10):a013300. doi: 10.1101/cshperspect.a013300. Cold Spring Harb Perspect Biol. 2012. PMID: 23028121 Free PMC article. Review.
-
Quantitative Models of Lipid Transfer and Membrane Contact Formation.Contact (Thousand Oaks). 2022;5:1-21. doi: 10.1177/25152564221096024. Epub 2022 May 4. Contact (Thousand Oaks). 2022. PMID: 36120532 Free PMC article.
-
Uncovering mechanisms of interorganelle lipid transport by enzymatic mass tagging.FEBS Lett. 2024 May;598(10):1292-1298. doi: 10.1002/1873-3468.14810. Epub 2024 Jan 24. FEBS Lett. 2024. PMID: 38268324 Review.
-
Lipid sorting: lipids do it on their own.Curr Biol. 2005 Jun 7;15(11):R421-3. doi: 10.1016/j.cub.2005.05.030. Curr Biol. 2005. PMID: 15936263 Review.
-
Lipid transfer proteins: the lipid commute via shuttles, bridges and tubes.Nat Rev Mol Cell Biol. 2019 Feb;20(2):85-101. doi: 10.1038/s41580-018-0071-5. Nat Rev Mol Cell Biol. 2019. PMID: 30337668 Review.
Cited by
-
Rab2A-mediated Golgi-lipid droplet interactions support very-low-density lipoprotein secretion in hepatocytes.EMBO J. 2024 Dec;43(24):6383-6409. doi: 10.1038/s44318-024-00288-x. Epub 2024 Nov 4. EMBO J. 2024. PMID: 39496977 Free PMC article.
-
"VTT"-domain proteins VMP1 and TMEM41B function in lipid homeostasis globally and locally as ER scramblases.Contact (Thousand Oaks). 2021 Jan 1;4:25152564211024494. doi: 10.1177/25152564211024494. Epub 2021 Jun 16. Contact (Thousand Oaks). 2021. PMID: 34447902 Free PMC article.
-
Lipid osmosis, membrane tension, and other mechanochemical driving forces of lipid flow.bioRxiv [Preprint]. 2024 Apr 28:2024.01.08.574656. doi: 10.1101/2024.01.08.574656. bioRxiv. 2024. Update in: Curr Opin Cell Biol. 2024 Jun;88:102377. doi: 10.1016/j.ceb.2024.102377 PMID: 38260424 Free PMC article. Updated. Preprint.
-
Asymmetric Lipid Transfer between Zwitterionic Vesicles by Nanoviscosity Measurements.Nanomaterials (Basel). 2021 Apr 22;11(5):1087. doi: 10.3390/nano11051087. Nanomaterials (Basel). 2021. PMID: 33922325 Free PMC article.
-
Pex30-like proteins function as adaptors at distinct ER membrane contact sites.J Cell Biol. 2021 Oct 4;220(10):e202103176. doi: 10.1083/jcb.202103176. Epub 2021 Aug 17. J Cell Biol. 2021. PMID: 34402813 Free PMC article.
References
-
- Area-Gomez, E., Del Carmen Lara Castillo M., Tambini M.D., Guardia-Laguarta C., de Groof A.J., Madra M., Ikenouchi J., Umeda M., Bird T.D., Sturley S.L., and Schon E.A.. 2012. Upregulated function of mitochondria-associated ER membranes in Alzheimer disease. EMBO J. 31:4106–4123. 10.1038/emboj.2012.202 - DOI - PMC - PubMed
Publication types
MeSH terms
Grants and funding
LinkOut - more resources
Full Text Sources
Other Literature Sources