Lipidome-based Targeting of STAT3-driven Breast Cancer Cells Using Poly-l-glutamic Acid-coated Layer-by-Layer Nanoparticles
- PMID: 33536189
- PMCID: PMC8026705
- DOI: 10.1158/1535-7163.MCT-20-0505
Lipidome-based Targeting of STAT3-driven Breast Cancer Cells Using Poly-l-glutamic Acid-coated Layer-by-Layer Nanoparticles
Abstract
The oncogenic transcription factor STAT3 is aberrantly activated in 70% of breast cancers, including nearly all triple-negative breast cancers (TNBCs). Because STAT3 is difficult to target directly, we considered whether metabolic changes driven by activated STAT3 could provide a therapeutic opportunity. We found that STAT3 prominently modulated several lipid classes, with most profound effects on N-acyl taurine and arachidonic acid, both of which are involved in plasma membrane remodeling. To exploit these metabolic changes therapeutically, we screened a library of layer-by-layer (LbL) nanoparticles (NPs) differing in the surface layer that modulates interactivity with the cell membrane. We found that poly-l-glutamic acid (PLE)-coated NPs bind to STAT3-transformed breast cancer cells with 50% greater efficiency than to nontransformed cells, and the heightened PLE-NP binding to TNBC cells was attenuated by STAT3 inhibition. This effect was also observed in densely packed three-dimensional breast cancer organoids. As STAT3-transformed cells show greater resistance to cytotoxic agents, we evaluated whether enhanced targeted delivery via PLE-NPs would provide a therapeutic advantage. We found that cisplatin-loaded PLE-NPs induced apoptosis of STAT3-driven cells at lower doses compared with both unencapsulated cisplatin and cisplatin-loaded nontargeted NPs. In addition, because radiation is commonly used in breast cancer treatment, and may alter cellular lipid distribution, we analyzed its effect on PLE-NP-cell binding. Irradiation of cells enhanced the STAT3-targeting properties of PLE-NPs in a dose-dependent manner, suggesting potential synergies between these therapeutic modalities. These findings suggest that cellular lipid changes driven by activated STAT3 may be exploited therapeutically using unique LbL NPs.
©2021 American Association for Cancer Research.
Conflict of interest statement
Figures
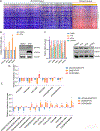
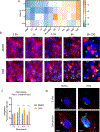
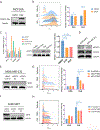
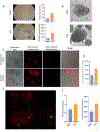
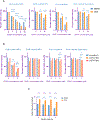
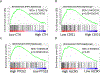
Similar articles
-
Aminoflavone-loaded EGFR-targeted unimolecular micelle nanoparticles exhibit anti-cancer effects in triple negative breast cancer.Biomaterials. 2016 Sep;101:20-31. doi: 10.1016/j.biomaterials.2016.05.041. Epub 2016 May 27. Biomaterials. 2016. PMID: 27267625 Free PMC article.
-
RNA-binding protein NONO contributes to cancer cell growth and confers drug resistance as a theranostic target in TNBC.Theranostics. 2020 Jul 2;10(18):7974-7992. doi: 10.7150/thno.45037. eCollection 2020. Theranostics. 2020. PMID: 32724453 Free PMC article.
-
Novel Galiellalactone Analogues Can Target STAT3 Phosphorylation and Cause Apoptosis in Triple-Negative Breast Cancer.Biomolecules. 2019 May 3;9(5):170. doi: 10.3390/biom9050170. Biomolecules. 2019. PMID: 31058868 Free PMC article.
-
Stress-induced EGF receptor signaling through STAT3 and tumor progression in triple-negative breast cancer.Mol Cell Endocrinol. 2017 Aug 15;451:24-30. doi: 10.1016/j.mce.2017.01.013. Epub 2017 Jan 12. Mol Cell Endocrinol. 2017. PMID: 28088463 Free PMC article. Review.
-
Dual inhibition of STAT1 and STAT3 activation downregulates expression of PD-L1 in human breast cancer cells.Expert Opin Ther Targets. 2018 Jun;22(6):547-557. doi: 10.1080/14728222.2018.1471137. Epub 2018 May 2. Expert Opin Ther Targets. 2018. PMID: 29702007 Review.
Cited by
-
Unraveling the intricate relationship between lipid metabolism and oncogenic signaling pathways.Front Cell Dev Biol. 2024 Jun 12;12:1399065. doi: 10.3389/fcell.2024.1399065. eCollection 2024. Front Cell Dev Biol. 2024. PMID: 38933330 Free PMC article. Review.
-
"Target-and-release" nanoparticles for effective immunotherapy of metastatic ovarian cancer.bioRxiv [Preprint]. 2024 Jul 7:2024.07.05.602135. doi: 10.1101/2024.07.05.602135. bioRxiv. 2024. PMID: 39005274 Free PMC article. Preprint.
-
Advances in Analyzing the Breast Cancer Lipidome and Its Relevance to Disease Progression and Treatment.J Mammary Gland Biol Neoplasia. 2021 Dec;26(4):399-417. doi: 10.1007/s10911-021-09505-3. Epub 2021 Dec 16. J Mammary Gland Biol Neoplasia. 2021. PMID: 34914014 Free PMC article. Review.
References
Publication types
MeSH terms
Substances
Grants and funding
LinkOut - more resources
Full Text Sources
Other Literature Sources
Research Materials
Miscellaneous