The Mechanism of NEDD8 Activation of CUL5 Ubiquitin E3 Ligases
- PMID: 33268465
- PMCID: PMC7950132
- DOI: 10.1074/mcp.RA120.002414
The Mechanism of NEDD8 Activation of CUL5 Ubiquitin E3 Ligases
Abstract
Cullin RING E3 ligases (CRLs) ubiquitylate hundreds of important cellular substrates. Here we have assembled and purified the Ankyrin repeat and SOCS Box protein 9 CUL5 RBX2 ligase (ASB9-CRL) in vitro and show how it ubiquitylates one of its substrates, CKB. CRLs occasionally collaborate with RING between RING E3 ligases (RBRLs), and indeed, mass spectrometry analysis showed that CKB is specifically ubiquitylated by the ASB9-CRL-ARIH2-UBE2L3 complex. Addition of other E2s such as UBE2R1 or UBE2D2 contributes to polyubiquitylation but does not alter the sites of CKB ubiquitylation. Hydrogen-deuterium exchange mass spectrometry (HDX-MS) analysis revealed that CUL5 neddylation allosterically exposes its ARIH2 binding site, promoting high-affinity binding, and it also sequesters the NEDD8 E2 (UBE2F) binding site on RBX2. Once bound, ARIH2 helices near the Ariadne domain active site are exposed, presumably relieving its autoinhibition. These results allow us to propose a model of how neddylation activates ASB-CRLs to ubiquitylate their substrates.
Keywords: hydrogen-deuterium exchange mass spectrometry; post-translational modifications; protein complex; ubiquitin ligase.
Copyright © 2021 The Authors. Published by Elsevier Inc. All rights reserved.
Conflict of interest statement
Conflicts of interest Authors declare no competing interests.
Figures
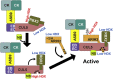
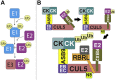
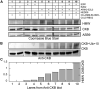
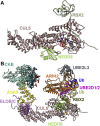
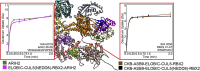
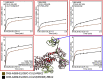
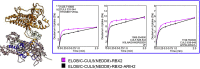
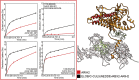
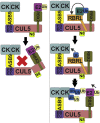
Similar articles
-
Biophysical studies on interactions and assembly of full-size E3 ubiquitin ligase: suppressor of cytokine signaling 2 (SOCS2)-elongin BC-cullin 5-ring box protein 2 (RBX2).J Biol Chem. 2015 Feb 13;290(7):4178-91. doi: 10.1074/jbc.M114.616664. Epub 2014 Dec 11. J Biol Chem. 2015. PMID: 25505247 Free PMC article.
-
CUL5-ARIH2 E3-E3 ubiquitin ligase structure reveals cullin-specific NEDD8 activation.Nat Chem Biol. 2021 Oct;17(10):1075-1083. doi: 10.1038/s41589-021-00858-8. Epub 2021 Sep 13. Nat Chem Biol. 2021. PMID: 34518685 Free PMC article.
-
Structure and dynamics of the ASB9 CUL-RING E3 Ligase.Nat Commun. 2020 Jun 8;11(1):2866. doi: 10.1038/s41467-020-16499-9. Nat Commun. 2020. PMID: 32513959 Free PMC article.
-
Cullin RING Ligase 5 (CRL-5): Neddylation Activation and Biological Functions.Adv Exp Med Biol. 2020;1217:261-283. doi: 10.1007/978-981-15-1025-0_16. Adv Exp Med Biol. 2020. PMID: 31898233 Review.
-
Cullin-RING Ubiquitin Ligase Regulatory Circuits: A Quarter Century Beyond the F-Box Hypothesis.Annu Rev Biochem. 2021 Jun 20;90:403-429. doi: 10.1146/annurev-biochem-090120-013613. Epub 2021 Apr 6. Annu Rev Biochem. 2021. PMID: 33823649 Free PMC article. Review.
Cited by
-
Cullin5 drives experimental asthma exacerbations by modulating alveolar macrophage antiviral immunity.Nat Commun. 2024 Jan 4;15(1):252. doi: 10.1038/s41467-023-44168-0. Nat Commun. 2024. PMID: 38177117 Free PMC article.
-
Hydrogen/Deuterium Exchange and Nuclear Magnetic Resonance Spectroscopy Reveal Dynamic Allostery on Multiple Time Scales in the Serine Protease Thrombin.Biochemistry. 2021 Nov 23;60(46):3441-3448. doi: 10.1021/acs.biochem.1c00277. Epub 2021 Jun 23. Biochemistry. 2021. PMID: 34159782 Free PMC article.
-
Targeting neddylation E2s: a novel therapeutic strategy in cancer.J Hematol Oncol. 2021 Apr 7;14(1):57. doi: 10.1186/s13045-021-01070-w. J Hematol Oncol. 2021. PMID: 33827629 Free PMC article. Review.
-
CLOCK inhibits the proliferation of porcine ovarian granulosa cells by targeting ASB9.J Anim Sci Biotechnol. 2023 Jun 7;14(1):82. doi: 10.1186/s40104-023-00884-7. J Anim Sci Biotechnol. 2023. PMID: 37280645 Free PMC article.
-
Noncanonical assembly, neddylation and chimeric cullin-RING/RBR ubiquitylation by the 1.8 MDa CUL9 E3 ligase complex.Nat Struct Mol Biol. 2024 Jul;31(7):1083-1094. doi: 10.1038/s41594-024-01257-y. Epub 2024 Apr 11. Nat Struct Mol Biol. 2024. PMID: 38605244 Free PMC article.
References
-
- Komander D., Rape M. The ubiquitin code. Annu. Rev. Biochem. 2012;81:203–229. - PubMed
-
- Akutsu M., Dikic I., Bremm A. Ubiquitin chain diversity at a glance. J. Cell Sci. 2016;129:875–880. - PubMed
-
- Ohtake F., Saeki Y., Ishido S., Kanno J., Tanaka K. The K48-K63 branched ubiquitin chain regulates NF-kB signaling. Mol. Cell. 2016;64:251–266. - PubMed
-
- Yau R., Rape M. The increasing complexity of the ubiquitin code. Nat. Cell Biol. 2016;18:579–586. - PubMed
Publication types
MeSH terms
Substances
Grants and funding
LinkOut - more resources
Full Text Sources
Other Literature Sources
Research Materials
Miscellaneous