Complete Mapping of Mutations to the SARS-CoV-2 Spike Receptor-Binding Domain that Escape Antibody Recognition
- PMID: 33259788
- PMCID: PMC7676316
- DOI: 10.1016/j.chom.2020.11.007
Complete Mapping of Mutations to the SARS-CoV-2 Spike Receptor-Binding Domain that Escape Antibody Recognition
Abstract
Antibodies targeting the SARS-CoV-2 spike receptor-binding domain (RBD) are being developed as therapeutics and are a major contributor to neutralizing antibody responses elicited by infection. Here, we describe a deep mutational scanning method to map how all amino-acid mutations in the RBD affect antibody binding and apply this method to 10 human monoclonal antibodies. The escape mutations cluster on several surfaces of the RBD that broadly correspond to structurally defined antibody epitopes. However, even antibodies targeting the same surface often have distinct escape mutations. The complete escape maps predict which mutations are selected during viral growth in the presence of single antibodies. They further enable the design of escape-resistant antibody cocktails-including cocktails of antibodies that compete for binding to the same RBD surface but have different escape mutations. Therefore, complete escape-mutation maps enable rational design of antibody therapeutics and assessment of the antigenic consequences of viral evolution.
Keywords: SARS-CoV-2; antibody escape; antigenic evolution; deep mutational scanning.
Copyright © 2020 The Authors. Published by Elsevier Inc. All rights reserved.
Conflict of interest statement
Declaration of Interests J.E.C. has served as a consultant for Sanofi, is on the Scientific Advisory Boards of CompuVax and Meissa Vaccines, is a recipient of previous unrelated grants from Moderna and Sanofi, and is a founder of IDBiologics. Vanderbilt University has applied for patents on SARS-CoV-2 antibodies. S.P.J.W. and P.W.R. have filed a disclosure with Washington University for recombinant VSV. The other authors declare no competing interests.
Figures
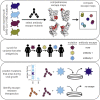
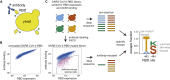
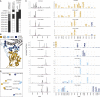
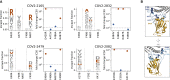
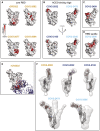
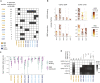
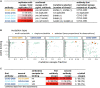
Update of
-
Complete mapping of mutations to the SARS-CoV-2 spike receptor-binding domain that escape antibody recognition.bioRxiv [Preprint]. 2020 Sep 28:2020.09.10.292078. doi: 10.1101/2020.09.10.292078. bioRxiv. 2020. Update in: Cell Host Microbe. 2021 Jan 13;29(1):44-57.e9. doi: 10.1016/j.chom.2020.11.007. PMID: 32935107 Free PMC article. Updated. Preprint.
Similar articles
-
Mapping mutations to the SARS-CoV-2 RBD that escape binding by different classes of antibodies.Nat Commun. 2021 Jul 7;12(1):4196. doi: 10.1038/s41467-021-24435-8. Nat Commun. 2021. PMID: 34234131 Free PMC article.
-
Comprehensive mapping of mutations in the SARS-CoV-2 receptor-binding domain that affect recognition by polyclonal human plasma antibodies.Cell Host Microbe. 2021 Mar 10;29(3):463-476.e6. doi: 10.1016/j.chom.2021.02.003. Epub 2021 Feb 8. Cell Host Microbe. 2021. PMID: 33592168 Free PMC article.
-
Prospective mapping of viral mutations that escape antibodies used to treat COVID-19.Science. 2021 Feb 19;371(6531):850-854. doi: 10.1126/science.abf9302. Epub 2021 Jan 25. Science. 2021. PMID: 33495308 Free PMC article.
-
Depressing time: Waiting, melancholia, and the psychoanalytic practice of care.In: Kirtsoglou E, Simpson B, editors. The Time of Anthropology: Studies of Contemporary Chronopolitics. Abingdon: Routledge; 2020. Chapter 5. In: Kirtsoglou E, Simpson B, editors. The Time of Anthropology: Studies of Contemporary Chronopolitics. Abingdon: Routledge; 2020. Chapter 5. PMID: 36137063 Free Books & Documents. Review.
-
Trends in Surgical and Nonsurgical Aesthetic Procedures: A 14-Year Analysis of the International Society of Aesthetic Plastic Surgery-ISAPS.Aesthetic Plast Surg. 2024 Oct;48(20):4217-4227. doi: 10.1007/s00266-024-04260-2. Epub 2024 Aug 5. Aesthetic Plast Surg. 2024. PMID: 39103642 Review.
Cited by
-
Recognition of the SARS-CoV-2 receptor binding domain by neutralizing antibodies.Biochem Biophys Res Commun. 2021 Jan 29;538:192-203. doi: 10.1016/j.bbrc.2020.10.012. Epub 2020 Oct 10. Biochem Biophys Res Commun. 2021. PMID: 33069360 Free PMC article. Review.
-
Deep mutational scans for ACE2 binding, RBD expression, and antibody escape in the SARS-CoV-2 Omicron BA.1 and BA.2 receptor-binding domains.PLoS Pathog. 2022 Nov 18;18(11):e1010951. doi: 10.1371/journal.ppat.1010951. eCollection 2022 Nov. PLoS Pathog. 2022. PMID: 36399443 Free PMC article.
-
One-shot identification of SARS-CoV-2 S RBD escape mutants using yeast screening.bioRxiv [Preprint]. 2021 Mar 15:2021.03.15.435309. doi: 10.1101/2021.03.15.435309. bioRxiv. 2021. Update in: Cell Rep. 2021 Aug 31;36(9):109627. doi: 10.1016/j.celrep.2021.109627. PMID: 33758848 Free PMC article. Updated. Preprint.
-
Mutational escape from the polyclonal antibody response to SARS-CoV-2 infection is largely shaped by a single class of antibodies.bioRxiv [Preprint]. 2021 Mar 18:2021.03.17.435863. doi: 10.1101/2021.03.17.435863. bioRxiv. 2021. Update in: Nat Commun. 2021 Jul 7;12(1):4196. doi: 10.1038/s41467-021-24435-8. PMID: 33758856 Free PMC article. Updated. Preprint.
-
Will New Variants Emerge after Delta and Omicron?Aging Dis. 2022 Oct 1;13(5):1317-1322. doi: 10.14336/AD.2022.0307. eCollection 2022 Oct 1. Aging Dis. 2022. PMID: 36186131 Free PMC article. No abstract available.
References
-
- Addetia A., Crawford K.H.D., Dingens A., Zhu H., Roychoudhury P., Huang M.-L., Jerome K.R., Bloom J.D., Greninger A.L. Neutralizing antibodies correlate with protection from SARS-CoV-2 in humans during a fishery vessel outbreak with high attack rate. J. Clin. Microbiol. 2020;58:e02107-20. - PMC - PubMed
-
- Barnes C.O., Jette C.A., Abernathy M.E., Dam K.A., Esswein S.R., Gristick H.B., Malyutin A.G., Sharaf N.G., Huey-Tubman K.E., Lee Y.E. Structural classification of neutralizing antibodies against the SARS-CoV-2 spike receptor-binding domain suggests vaccine and therapeutic strategies. bioRxiv. 2020 2020.08.30.273920.
MeSH terms
Substances
Grants and funding
LinkOut - more resources
Full Text Sources
Other Literature Sources
Research Materials
Miscellaneous