Integrity of the Antiviral STING-mediated DNA Sensing in Tumor Cells Is Required to Sustain the Immunotherapeutic Efficacy of Herpes Simplex Oncolytic Virus
- PMID: 33213060
- PMCID: PMC7698602
- DOI: 10.3390/cancers12113407
Integrity of the Antiviral STING-mediated DNA Sensing in Tumor Cells Is Required to Sustain the Immunotherapeutic Efficacy of Herpes Simplex Oncolytic Virus
Abstract
The dichotomic contribution of cancer cell lysis and tumor immunogenicity is considered essential for effective oncovirotherapy, suggesting that the innate antiviral immune response is a hurdle for efficacy of oncolytic viruses. However, emerging evidence is resizing this view. By sensing cytosolic DNA, the cyclic GMP-AMP synthase (cGAS) and stimulator of interferon genes (STING) axis can both counteract viral spread and contribute to the elicitation of adaptive immunity via type I interferon responses. In this paper, we analyzed the tumor-resident function of Sting-mediated DNA sensing in a combined approach of oncovirotherapy and PD-1 immune checkpoint blockade, in an immunocompetent murine model. While supporting increased lytic potential by oncolytic HER2-retargeted HSV-1 in vitro and in vivo, Sting-knockout tumors showed molecular signatures of an immunosuppressive tumor microenvironment. These signatures were correspondingly associated with ineffectiveness of the combination therapy in a model of established tumors. Results suggest that the impairment in antiviral response of Sting-knockout tumors, while favoring viral replication, is not able to elicit an adequate immunotherapeutic effect, due to lack of immunogenic cell death and the inability of Sting-knockout cancer cells to promote anti-tumor adaptive immune responses. Accordingly, we propose that antiviral, tumor-resident Sting provides fundamental contributions to immunotherapeutic efficacy of oncolytic viruses.
Keywords: HSV-1; Herpes simplex; MB21D; RNA profiling; STING knockout; TMEM173; immunogenic cell death; oncolytic virus.
Conflict of interest statement
The Authors, Alfredo Nicosia and Elisa Scarselli are Founders and shareholders of Nouscom S.R.L. Emanuele Sasso, Gabriella Cotugno, Anna Morena D’Alise, Guido Leoni, Maria De Lucia, Francesca Langone are employees of Nouscom S.R.L. All the other Authors declare no competing interests.
Figures
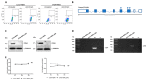
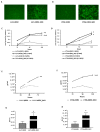
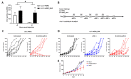
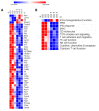
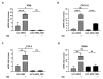
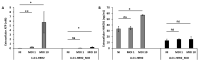
Similar articles
-
Diminished Innate Antiviral Response to Adenovirus Vectors in cGAS/STING-Deficient Mice Minimally Impacts Adaptive Immunity.J Virol. 2016 Jun 10;90(13):5915-27. doi: 10.1128/JVI.00500-16. Print 2016 Jul 1. J Virol. 2016. PMID: 27076643 Free PMC article.
-
Oncolytic virus immunotherapy induces immunogenic cell death and overcomes STING deficiency in melanoma.Oncoimmunology. 2019 Apr 7;8(7):1591875. doi: 10.1080/2162402X.2019.1591875. eCollection 2019. Oncoimmunology. 2019. PMID: 31143509 Free PMC article.
-
Herpes Simplex Virus 1 Serine Protease VP24 Blocks the DNA-Sensing Signal Pathway by Abrogating Activation of Interferon Regulatory Factor 3.J Virol. 2016 May 27;90(12):5824-5829. doi: 10.1128/JVI.00186-16. Print 2016 Jun 15. J Virol. 2016. PMID: 27076640 Free PMC article.
-
Role of the cGAS-STING pathway in cancer development and oncotherapeutic approaches.EMBO Rep. 2018 Dec;19(12):e46935. doi: 10.15252/embr.201846935. Epub 2018 Nov 16. EMBO Rep. 2018. PMID: 30446584 Free PMC article. Review.
-
The Antiviral Apparatus: STING and Oncolytic Virus Restriction.Mol Ther Oncolytics. 2019 Mar 20;13:7-13. doi: 10.1016/j.omto.2019.02.002. eCollection 2019 Jun 28. Mol Ther Oncolytics. 2019. PMID: 30989105 Free PMC article. Review.
Cited by
-
Oncolytic Viro-Immunotherapy: An Emerging Option in the Treatment of Gliomas.Front Immunol. 2021 Oct 5;12:721830. doi: 10.3389/fimmu.2021.721830. eCollection 2021. Front Immunol. 2021. PMID: 34675919 Free PMC article. Review.
-
The Association of Human Herpesviruses with Malignant Brain Tumor Pathology and Therapy: Two Sides of a Coin.Int J Mol Sci. 2021 Feb 24;22(5):2250. doi: 10.3390/ijms22052250. Int J Mol Sci. 2021. PMID: 33668202 Free PMC article. Review.
-
Growth, Purification, and Titration of Oncolytic Herpes Simplex Virus.J Vis Exp. 2021 May 13;(171):10.3791/62677. doi: 10.3791/62677. J Vis Exp. 2021. PMID: 34057449 Free PMC article.
-
USP18 and USP20 restrict oHSV-1 replication in resistant human oral squamous carcinoma cell line SCC9 and affect the viability of SCC9 cells.Mol Ther Oncolytics. 2021 Nov 11;23:477-487. doi: 10.1016/j.omto.2021.11.004. eCollection 2021 Dec 17. Mol Ther Oncolytics. 2021. PMID: 34901390 Free PMC article.
-
Oncolytic HSV: Underpinnings of Tumor Susceptibility.Viruses. 2021 Jul 20;13(7):1408. doi: 10.3390/v13071408. Viruses. 2021. PMID: 34372614 Free PMC article. Review.
References
-
- Ribas A., Dummer R., Puzanov I., Vander Walde A., Andtbacka R.H.I., Michielin O., Olszanksi A.J., Malvehy J., Cebon J., Fernandez E., et al. Oncolytic Virotherapy Promotes Intratumoral T Cell Infiltration and Improves Anti-PD-1 Immunotherapy. Cell. 2017;170:1109–1119. doi: 10.1016/j.cell.2017.08.027. - DOI - PMC - PubMed
Grants and funding
LinkOut - more resources
Full Text Sources
Research Materials
Miscellaneous