Optimized gene engineering of murine CAR-T cells reveals the beneficial effects of IL-15 coexpression
- PMID: 33156338
- PMCID: PMC7653685
- DOI: 10.1084/jem.20192203
Optimized gene engineering of murine CAR-T cells reveals the beneficial effects of IL-15 coexpression
Abstract
Limited clinical benefit has been demonstrated for chimeric antigen receptor (CAR) therapy of solid tumors, but coengineering strategies to generate so-called fourth-generation (4G) CAR-T cells are advancing toward overcoming barriers in the tumor microenvironment (TME) for improved responses. In large part due to technical challenges, there are relatively few preclinical CAR therapy studies in immunocompetent, syngeneic tumor-bearing mice. Here, we describe optimized methods for the efficient retroviral transduction and expansion of murine T lymphocytes of a predominantly central memory T cell (TCM cell) phenotype. We present a bicistronic retroviral vector encoding both a tumor vasculature-targeted CAR and murine interleukin-15 (mIL-15), conferring enhanced effector functions, engraftment, tumor control, and TME reprogramming, including NK cell activation and reduced presence of M2 macrophages. The 4G-CAR-T cells coexpressing mIL-15 were further characterized by up-regulation of the antiapoptotic marker Bcl-2 and lower cell-surface expression of the inhibitory receptor PD-1. Overall, this work introduces robust tools for the development and evaluation of 4G-CAR-T cells in immunocompetent mice, an important step toward the acceleration of effective therapies reaching the clinic.
© 2020 Lanitis et al.
Conflict of interest statement
Disclosures: G. Coukos reported grants from Celgene, Boehringer-Ingelheim, Roche, BMS, Iovance Therapeutics, and Kite Pharma; personal fees from Genentech, Roche, BMS, AstraZeneca, NextCure, Geneos Tx, and Sanofi/Aventis outside the submitted work; and had patents in the domain of antibodies and vaccines targeting the tumor vasculature as well as technologies related to T cell expansion and engineering for T cell therapy. G. Coukos holds patents around TEM1 antibodies and receives royalties from the University of Pennsylvania. No other disclosures were reported.
Figures
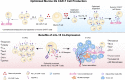
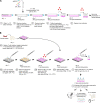
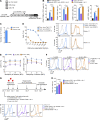
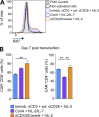
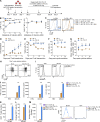
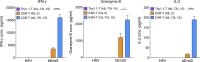
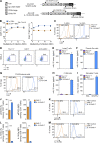
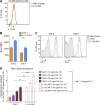
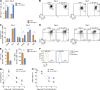
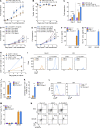
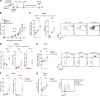
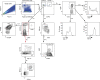
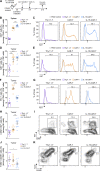
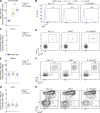
Similar articles
-
Generation of Chimeric Antigen Receptor T Cells Using Gammaretroviral Vectors.Methods Mol Biol. 2020;2086:119-130. doi: 10.1007/978-1-0716-0146-4_8. Methods Mol Biol. 2020. PMID: 31707671
-
Use of Cell and Genome Modification Technologies to Generate Improved "Off-the-Shelf" CAR T and CAR NK Cells.Front Immunol. 2020 Aug 7;11:1965. doi: 10.3389/fimmu.2020.01965. eCollection 2020. Front Immunol. 2020. PMID: 32903482 Free PMC article. Review.
-
A metabolic switch to memory CAR T cells: Implications for cancer treatment.Cancer Lett. 2021 Mar 1;500:107-118. doi: 10.1016/j.canlet.2020.12.004. Epub 2020 Dec 5. Cancer Lett. 2021. PMID: 33290868
-
CAR T Cells Redirected to CD44v6 Control Tumor Growth in Lung and Ovary Adenocarcinoma Bearing Mice.Front Immunol. 2020 Feb 4;11:99. doi: 10.3389/fimmu.2020.00099. eCollection 2020. Front Immunol. 2020. PMID: 32117253 Free PMC article.
-
Engineering strategies to safely drive CAR T-cells into the future.Front Immunol. 2024 Jun 19;15:1411393. doi: 10.3389/fimmu.2024.1411393. eCollection 2024. Front Immunol. 2024. PMID: 38962002 Free PMC article. Review.
Cited by
-
Transgenic Expression of IL15 Retains CD123-Redirected T Cells in a Less Differentiated State Resulting in Improved Anti-AML Activity in Autologous AML PDX Models.Front Immunol. 2022 May 9;13:880108. doi: 10.3389/fimmu.2022.880108. eCollection 2022. Front Immunol. 2022. PMID: 35615350 Free PMC article.
-
Heterodimeric IL-15 in Cancer Immunotherapy.Cancers (Basel). 2021 Feb 17;13(4):837. doi: 10.3390/cancers13040837. Cancers (Basel). 2021. PMID: 33671252 Free PMC article. Review.
-
CAR-T cell combination therapy: the next revolution in cancer treatment.Cancer Cell Int. 2022 Nov 24;22(1):365. doi: 10.1186/s12935-022-02778-6. Cancer Cell Int. 2022. PMID: 36419058 Free PMC article. Review.
-
Fc receptor-like 5 (FCRL5)-directed CAR-T cells exhibit antitumor activity against multiple myeloma.Signal Transduct Target Ther. 2024 Jan 12;9(1):16. doi: 10.1038/s41392-023-01702-2. Signal Transduct Target Ther. 2024. PMID: 38212320 Free PMC article.
-
Secretory co-factors in next-generation cellular therapies for cancer.Front Immunol. 2022 Aug 18;13:907022. doi: 10.3389/fimmu.2022.907022. eCollection 2022. Front Immunol. 2022. PMID: 36059449 Free PMC article. Review.
References
-
- Ahmadzadeh, M., Johnson L.A., Heemskerk B., Wunderlich J.R., Dudley M.E., White D.E., and Rosenberg S.A.. 2009. Tumor antigen-specific CD8 T cells infiltrating the tumor express high levels of PD-1 and are functionally impaired. Blood. 114:1537–1544. 10.1182/blood-2008-12-195792 - DOI - PMC - PubMed
-
- Avanzi, M.P., Yeku O., Li X., Wijewarnasuriya D.P., van Leeuwen D.G., Cheung K., Park H., Purdon T.J., Daniyan A.F., Spitzer M.H., and Brentjens R.J.. 2018. Engineered Tumor-Targeted T Cells Mediate Enhanced Anti-Tumor Efficacy Both Directly and through Activation of the Endogenous Immune System. Cell Rep. 23:2130–2141. 10.1016/j.celrep.2018.04.051 - DOI - PMC - PubMed
Publication types
MeSH terms
Substances
LinkOut - more resources
Full Text Sources
Other Literature Sources