A High-Fat Diet Increases Gut Microbiota Biodiversity and Energy Expenditure Due to Nutrient Difference
- PMID: 33092019
- PMCID: PMC7589760
- DOI: 10.3390/nu12103197
A High-Fat Diet Increases Gut Microbiota Biodiversity and Energy Expenditure Due to Nutrient Difference
Abstract
A high-fat diet (HFD) can easily induce obesity and change the gut microbiota and its metabolites. However, studies on the effects of high-fat diets on the host have drawn inconsistent results. In this study, the unexpected results showed that the refined HFD increased gut microbiota diversity and short-chain fatty acids (SCFAs), causing an increase in energy metabolism. Further analysis revealed these changes were caused by the different fiber content in these two diets. Male C57BL/6J mice (4-5 weeks old) were fed either HFD or refined low-fat diet (LFD) for 14 weeks. The metabolic rates, thermogenesis, gut microbiome, and intestinal SCFAs were tested. The HFD triggered obesity and disturbed glucose homeostasis. Mice fed HFD ingested more fiber than mice fed LFD (p < 0.0001), causing higher intestinal SCFA concentrations related to the increased abundances of specific bacteria in the HFD group. Also, the HFD increased metabolic heat and up-regulated thermogenesis genes uncoupling protein 1(Ucp-1), peroxisome proliferator-activated receptor-γ coactivator-1α (Pgc-1α) expression in the brown adipose tissue (BAT). It was revealed by 16S rRNA gene sequencing that the HFD increased gut microbial diversity, which enriched Desulfovibrionaceae, Rikenellaceae RC9 gut group, and Mucispirillum, meanwhile, reduced the abundance of Lactobacillus, Bifidobacterium, Akkermansia, Faecalibaculum, and Blautia. The predicted metabolic pathways indicated HFD increased the gene expression of non-absorbed carbohydrate metabolism pathways, as well as the risks of colonization of intestinal pathogens and inflammation. In conclusion, the HFD was obesogenic in male C57BL/6J mice, and increased fiber intake from the HFD drove an increase in gut microbiota diversity, SCFAs, and energy expenditure. Meanwhile, the differences in specific nutrient intake can dissociate broad changes in energy expenditure, gut microbiota, and its metabolites from obesity, raising doubts in the previous studies. Therefore, it is necessary to consider whether differences in specific nutrient intake will interfere with the results of the experiments.
Keywords: SCFAs; energy expenditure; fiber; glucose homeostasis; gut microbiota; high-fat diet; low-fat diet; obesity.
Conflict of interest statement
The authors declare no conflict of interest.
Figures
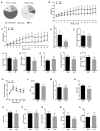
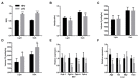
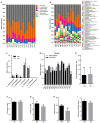
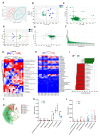
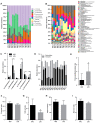
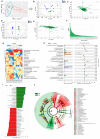
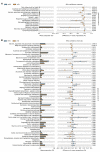
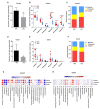
Similar articles
-
Bifidobacterium adolescentis Isolated from Different Hosts Modifies the Intestinal Microbiota and Displays Differential Metabolic and Immunomodulatory Properties in Mice Fed a High-Fat Diet.Nutrients. 2021 Mar 21;13(3):1017. doi: 10.3390/nu13031017. Nutrients. 2021. PMID: 33801119 Free PMC article.
-
Dietary α-lactalbumin alters energy balance, gut microbiota composition and intestinal nutrient transporter expression in high-fat diet-fed mice.Br J Nutr. 2019 May;121(10):1097-1107. doi: 10.1017/S0007114519000461. Epub 2019 Mar 5. Br J Nutr. 2019. PMID: 30834845
-
Functional Fiber Reduces Mice Obesity by Regulating Intestinal Microbiota.Nutrients. 2022 Jun 28;14(13):2676. doi: 10.3390/nu14132676. Nutrients. 2022. PMID: 35807856 Free PMC article.
-
Short-chain fatty acids: bridges between diet, gut microbiota, and health.J Gastroenterol Hepatol. 2024 Sep;39(9):1728-1736. doi: 10.1111/jgh.16619. Epub 2024 May 23. J Gastroenterol Hepatol. 2024. PMID: 38780349 Review.
-
Balancing Act: Exploring the Gut Microbiota-Brown Adipose Tissue Axis in PCOS Pathogenesis and Therapeutic Frontiers.Front Biosci (Landmark Ed). 2024 May 30;29(6):208. doi: 10.31083/j.fbl2906208. Front Biosci (Landmark Ed). 2024. PMID: 38940030 Review.
Cited by
-
Gut Dysbiosis Shaped by Cocoa Butter-Based Sucrose-Free HFD Leads to Steatohepatitis, and Insulin Resistance in Mice.Nutrients. 2024 Jun 18;16(12):1929. doi: 10.3390/nu16121929. Nutrients. 2024. PMID: 38931284 Free PMC article.
-
Extracted yam bean (Pachyrhizus erosus (L.) Urb.) fiber counteracts adiposity, insulin resistance, and inflammation while modulating gut microbiota composition in mice fed with a high-fat diet.Res Pharm Sci. 2022 Sep 8;17(5):558-571. doi: 10.4103/1735-5362.355213. eCollection 2022 Oct. Res Pharm Sci. 2022. PMID: 36386490 Free PMC article.
-
Effects of different foods and cooking methods on the gut microbiota: an in vitro approach.Front Microbiol. 2024 Jan 8;14:1334623. doi: 10.3389/fmicb.2023.1334623. eCollection 2023. Front Microbiol. 2024. PMID: 38260868 Free PMC article.
-
The gut microbiota during the progression of atherosclerosis in the perimenopausal period shows specific compositional changes and significant correlations with circulating lipid metabolites.Gut Microbes. 2021 Jan-Dec;13(1):1-27. doi: 10.1080/19490976.2021.1880220. Gut Microbes. 2021. PMID: 33691599 Free PMC article.
-
The effects of a set amount of regular maternal exercise during pregnancy on gut microbiota are diet-dependent in mice and do not cause significant diversity changes.PeerJ. 2022 Dec 2;10:e14459. doi: 10.7717/peerj.14459. eCollection 2022. PeerJ. 2022. PMID: 36518263 Free PMC article.
References
Publication types
MeSH terms
Substances
Grants and funding
LinkOut - more resources
Full Text Sources
Research Materials