Parthenolide promotes the repair of spinal cord injury by modulating M1/M2 polarization via the NF-κB and STAT 1/3 signaling pathway
- PMID: 33083018
- PMCID: PMC7538575
- DOI: 10.1038/s41420-020-00333-8
Parthenolide promotes the repair of spinal cord injury by modulating M1/M2 polarization via the NF-κB and STAT 1/3 signaling pathway
Abstract
Spinal cord injury (SCI) is a severe neurological disease; however, there is no effective treatment for spinal cord injury. Neuroinflammation involves the activation of resident microglia and the infiltration of macrophages is the major pathogenesis of SCI secondary injury and considered to be the therapeutic target of SCI. Parthenolide (PN) has been reported to exert anti-inflammatory effects in fever, migraines, arthritis, and superficial inflammation; however, the role of PN in SCI therapeutics has not been clarified. In this study, we showed that PN could improve the functional recovery of spinal cord in mice as revealed by increased BMS scores and decreased cavity of spinal cord injury in vivo. Immunofluorescence staining experiments confirmed that PN could promote axonal regeneration, increase myelin reconstitution, reduce chondroitin sulfate formation, inhibit scar hyperplasia, suppress the activation of A1 neurotoxic reactive astrocytes and facilitate shift from M1 to M2 polarization of microglia/macrophages. To verify how PN exerts its effects on microglia/macrophages polarization, we performed the mechanism study in vitro in microglia cell line BV-2. PN could significantly reduce M1 polarization in BV2 cells and partially rescue the decrease in the expression of M2 phenotype markers of microglia/macrophage induced by LPS, but no significant effect on M2 polarization stimulated with IL-4 was observed. Further study demonstrated PN inhibited NF-κB signal pathway directly or indirectly, and suppressed activation of signal transducer and activator of transcription 1 or 3 (STAT1/3) via reducing the expression of HDAC1 and subsequently increasing the levels of STAT1/3 acetylation. Overall, our study illustrated that PN may be a promising strategy for traumatic SCI.
Keywords: Microglia; Spinal cord diseases.
© The Author(s) 2020.
Conflict of interest statement
Conflict of interestThe authors declare that they have no conflict of interest.
Figures
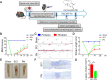
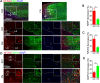
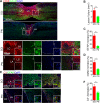
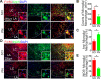
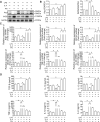
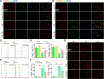
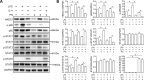
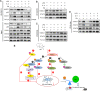
Similar articles
-
Salidroside attenuates neuroinflammation and improves functional recovery after spinal cord injury through microglia polarization regulation.J Cell Mol Med. 2018 Feb;22(2):1148-1166. doi: 10.1111/jcmm.13368. Epub 2017 Nov 17. J Cell Mol Med. 2018. PMID: 29148269 Free PMC article.
-
Valproic acid attenuates traumatic spinal cord injury-induced inflammation via STAT1 and NF-κB pathway dependent of HDAC3.J Neuroinflammation. 2018 May 18;15(1):150. doi: 10.1186/s12974-018-1193-6. J Neuroinflammation. 2018. PMID: 29776446 Free PMC article.
-
Curcumin-activated Olfactory Ensheathing Cells Improve Functional Recovery After Spinal Cord Injury by Modulating Microglia Polarization Through APOE/TREM2/NF-κB Signaling Pathway.J Neuroimmune Pharmacol. 2023 Sep;18(3):476-494. doi: 10.1007/s11481-023-10081-y. Epub 2023 Sep 2. J Neuroimmune Pharmacol. 2023. PMID: 37658943 Free PMC article.
-
Research Progress on Treating Spinal Cord Injury by Modulating the Phenotype of Microglia.J Integr Neurosci. 2024 Sep 19;23(9):171. doi: 10.31083/j.jin2309171. J Integr Neurosci. 2024. PMID: 39344231 Review.
-
Macrophage polarization in spinal cord injury repair and the possible role of microRNAs: A review.Heliyon. 2023 Nov 27;9(12):e22914. doi: 10.1016/j.heliyon.2023.e22914. eCollection 2023 Dec. Heliyon. 2023. PMID: 38125535 Free PMC article. Review.
Cited by
-
MFG-E8 regulated by miR-99b-5p protects against osteoarthritis by targeting chondrocyte senescence and macrophage reprogramming via the NF-κB pathway.Cell Death Dis. 2021 May 25;12(6):533. doi: 10.1038/s41419-021-03800-x. Cell Death Dis. 2021. PMID: 34031369 Free PMC article.
-
Molecular Mechanisms and Clinical Application of Multipotent Stem Cells for Spinal Cord Injury.Cells. 2022 Dec 28;12(1):120. doi: 10.3390/cells12010120. Cells. 2022. PMID: 36611914 Free PMC article. Review.
-
Neuroprotective Effects of the Pannexin-1 Channel Inhibitor: Probenecid on Spinal Cord Injury in Rats.Front Mol Neurosci. 2022 May 19;15:848185. doi: 10.3389/fnmol.2022.848185. eCollection 2022. Front Mol Neurosci. 2022. PMID: 35663270 Free PMC article.
-
Glia Signaling and Brain Microenvironment in Migraine.Mol Neurobiol. 2023 Jul;60(7):3911-3934. doi: 10.1007/s12035-023-03300-3. Epub 2023 Mar 30. Mol Neurobiol. 2023. PMID: 36995514 Review.
-
Modified exosomal SIRPα variants alleviate white matter injury after intracerebral hemorrhage via microglia/macrophages.Biomater Res. 2022 Nov 26;26(1):67. doi: 10.1186/s40824-022-00311-4. Biomater Res. 2022. PMID: 36435797 Free PMC article.
References
LinkOut - more resources
Full Text Sources
Research Materials
Miscellaneous