Accelerated degradation of HAP/PLLA bone scaffold by PGA blending facilitates bioactivity and osteoconductivity
- PMID: 32995675
- PMCID: PMC7493133
- DOI: 10.1016/j.bioactmat.2020.09.001
Accelerated degradation of HAP/PLLA bone scaffold by PGA blending facilitates bioactivity and osteoconductivity
Abstract
The incorporation of hydroxyapatite (HAP) into poly-l-lactic acid (PLLA) matrix serving as bone scaffold is expected to exhibit bioactivity and osteoconductivity to those of the living bone. While too low degradation rate of HAP/PLLA scaffold hinders the activity because the embedded HAP in the PLLA matrix is difficult to contact and exchange ions with body fluid. In this study, biodegradable polymer poly (glycolic acid) (PGA) was blended into the HAP/PLLA scaffold fabricated by laser 3D printing to accelerate the degradation. The results indicated that the incorporation of PGA enhanced the degradation rate of scaffold as indicated by the weight loss increasing from 3.3% to 25.0% after immersion for 28 days, owing to the degradation of high hydrophilic PGA and the subsequent accelerated hydrolysis of PLLA chains. Moreover, a lot of pores produced by the degradation of the scaffold promoted the exposure of HAP from the matrix, which not only activated the deposition of bone like apatite on scaffold but also accelerated apatite growth. Cytocompatibility tests exhibited a good osteoblast adhesion, spreading and proliferation, suggesting the scaffold provided a suitable environment for cell cultivation. Furthermore, the scaffold displayed excellent bone defect repair capacity with the formation of abundant new bone tissue and blood vessel tissue, and both ends of defect region were bridged after 8 weeks of implantation.
Keywords: Bone regeneration; Degradation; HAP/PLLA; PGA; Scaffold.
© 2020 [The Author/The Authors].
Conflict of interest statement
The authors declare that they have no known competing financial interests or personal relationships that could have appeared to influence the work reported in this paper.
Figures
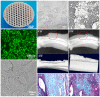
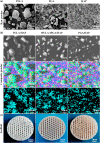
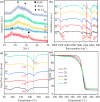
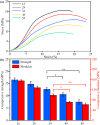
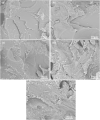
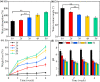
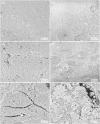
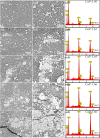
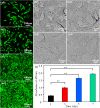
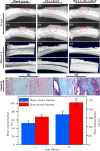
Similar articles
-
Construction of a stereocomplex between poly(D-lactide) grafted hydroxyapatite and poly(L-lactide): toward a bioactive composite scaffold with enhanced interfacial bonding.J Mater Chem B. 2022 Jan 5;10(2):214-223. doi: 10.1039/d1tb02111g. J Mater Chem B. 2022. PMID: 34927656
-
In Situ Generation of Hydroxyapatite on Biopolymer Particles for Fabrication of Bone Scaffolds Owning Bioactivity.ACS Appl Mater Interfaces. 2020 Oct 14;12(41):46743-46755. doi: 10.1021/acsami.0c13768. Epub 2020 Sep 30. ACS Appl Mater Interfaces. 2020. PMID: 32940994
-
In situ synthesis of hydroxyapatite nanorods on graphene oxide nanosheets and their reinforcement in biopolymer scaffold.J Adv Res. 2021 Apr 5;35:13-24. doi: 10.1016/j.jare.2021.03.009. eCollection 2022 Jan. J Adv Res. 2021. PMID: 35024192 Free PMC article.
-
Phosphonic Acid Coupling Agent Modification of HAP Nanoparticles: Interfacial Effects in PLLA/HAP Bone Scaffold.Polymers (Basel). 2020 Jan 13;12(1):199. doi: 10.3390/polym12010199. Polymers (Basel). 2020. PMID: 31940986 Free PMC article.
-
Towards resorbable 3D-printed scaffolds for craniofacial bone regeneration.Orthod Craniofac Res. 2023 Dec;26 Suppl 1:188-195. doi: 10.1111/ocr.12645. Epub 2023 Mar 13. Orthod Craniofac Res. 2023. PMID: 36866957 Review.
Cited by
-
Advances in electroactive biomaterials: Through the lens of electrical stimulation promoting bone regeneration strategy.J Orthop Translat. 2024 Jun 27;47:191-206. doi: 10.1016/j.jot.2024.06.009. eCollection 2024 Jul. J Orthop Translat. 2024. PMID: 39040489 Free PMC article. Review.
-
Advances in Barrier Membranes for Guided Bone Regeneration Techniques.Front Bioeng Biotechnol. 2022 Jun 22;10:921576. doi: 10.3389/fbioe.2022.921576. eCollection 2022. Front Bioeng Biotechnol. 2022. PMID: 35814003 Free PMC article. Review.
-
Recent advancement in 3-D printing: nanocomposites with added functionality.Prog Addit Manuf. 2022;7(2):325-350. doi: 10.1007/s40964-021-00232-z. Epub 2021 Oct 30. Prog Addit Manuf. 2022. PMID: 38624631 Free PMC article. Review.
-
Biomaterial-assisted tumor therapy: A brief review of hydroxyapatite nanoparticles and its composites used in bone tumors therapy.Front Bioeng Biotechnol. 2023 Apr 7;11:1167474. doi: 10.3389/fbioe.2023.1167474. eCollection 2023. Front Bioeng Biotechnol. 2023. PMID: 37091350 Free PMC article. Review.
-
Preparation and characterization of different micro/nano structures on the surface of bredigite scaffolds.Sci Rep. 2023 Jun 5;13(1):9072. doi: 10.1038/s41598-023-36382-z. Sci Rep. 2023. PMID: 37277439 Free PMC article.
References
-
- Zhou Y.Y., Li S., Wang D.L., Han X., Lei X. Fabrication of collagen/HAP electrospun scaffolds with perfect HAP nanorods along nanofiber orientation. Sci. Adv. Mater. 2018;10:930–936.
-
- Kim J.Y., Ahn G., Kim C., Lee J.S., Lee I.G., An S.H. Synergistic effects of beta tri-calcium phosphate and porcine-derived decellularized bone extracellular matrix in 3D-printed polycaprolactone scaffold on bone regeneration. Macromol. Biosci. 2018;18:1800025. - PubMed
-
- Lee S., Joshi M.K., Tiwari A.P., Maharjan B., Kim K.S., Yun Y.H. Lactic acid assisted fabrication of bioactive three-dimensional PLLA/β-TCP fibrous scaffold for biomedical application. Chem. Eng. J. 2018;347:771–781.
LinkOut - more resources
Full Text Sources
Other Literature Sources