TRPV4-Mediated Regulation of the Blood Brain Barrier Is Abolished During Inflammation
- PMID: 32974355
- PMCID: PMC7481434
- DOI: 10.3389/fcell.2020.00849
TRPV4-Mediated Regulation of the Blood Brain Barrier Is Abolished During Inflammation
Abstract
Blood-brain barrier (BBB) dysfunction is critically involved in determining the extent of several central nervous systems (CNS) pathologies and here in particular neuroinflammatory conditions. Inhibiting BBB breakdown could reduce the level of vasogenic edema and the number of immune cells invading the CNS, thereby counteracting neuronal injury. Transient receptor potential (TRP) channels have an important role as environmental sensors and constitute attractive therapeutic targets that are involved in calcium homeostasis during pathologies of the CNS. Transient receptor potential vanilloid 4 (TRPV4) is a calcium permeable, non-selective cation channel highly expressed in endothelial cells. As it is involved in the regulation of the blood brain barrier permeability and consequently cerebral edema formation, we anticipated a regulatory role of TRPV4 in CNS inflammation and subsequent neuronal damage. Here, we detected an increase in transendothelial resistance in mouse brain microvascular endothelial cells (MbMECs) after treatment with a selective TRPV4 inhibitor. However, this effect was abolished after the addition of IFNγ and TNFα indicating that inflammatory conditions override TRPV4-mediated permeability. Accordingly, we did not observe a protection of Trpv4-deficient mice when compared to wildtype controls in a preclinical model of multiple sclerosis, experimental autoimmune encephalomyelitis (EAE), and no differences in infarct sizes following transient middle cerebral artery occlusion (tMCAO), the experimental stroke model, which leads to an acute postischemic inflammatory response. Furthermore, Evans Blue injections did not show differences in alterations of the blood brain barrier (BBB) permeability between genotypes in both animal models. Together, TRPV4 does not regulate brain microvascular endothelial permeability under inflammation.
Keywords: TRPV4; blood brain barrier; experimental autoimmune encephalomyelitis; stroke; transendothelial resistance.
Copyright © 2020 Rosenkranz, Shaposhnykov, Schnapauff, Epping, Vieira, Heidermann, Schattling, Tsvilovskyy, Liedtke, Meuth, Freichel, Gelderblom and Friese.
Figures
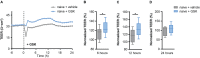
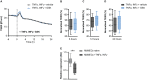
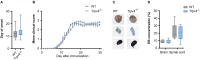
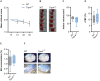
Similar articles
-
Inflammation-induced TRPV4 channels exacerbate blood-brain barrier dysfunction in multiple sclerosis.J Neuroinflammation. 2024 Mar 23;21(1):72. doi: 10.1186/s12974-024-03069-9. J Neuroinflammation. 2024. PMID: 38521959 Free PMC article.
-
Pharmacological Inhibition of Transient Receptor Potential Vanilloid 4 Reduces Vasogenic Edema after Traumatic Brain Injury in Mice.Biol Pharm Bull. 2021;44(11):1759-1766. doi: 10.1248/bpb.b21-00512. Biol Pharm Bull. 2021. PMID: 34719652
-
TRPV4 Blockade Preserves the Blood-Brain Barrier by Inhibiting Stress Fiber Formation in a Rat Model of Intracerebral Hemorrhage.Front Mol Neurosci. 2018 Mar 27;11:97. doi: 10.3389/fnmol.2018.00097. eCollection 2018. Front Mol Neurosci. 2018. PMID: 29636662 Free PMC article.
-
Structural pathways for macromolecular and cellular transport across the blood-brain barrier during inflammatory conditions. Review.Histol Histopathol. 2004 Apr;19(2):535-64. doi: 10.14670/HH-19.535. Histol Histopathol. 2004. PMID: 15024715 Review.
-
Role of Transient Receptor Potential Vanilloid 4 in Vascular Function.Front Mol Biosci. 2021 Apr 26;8:677661. doi: 10.3389/fmolb.2021.677661. eCollection 2021. Front Mol Biosci. 2021. PMID: 33981725 Free PMC article. Review.
Cited by
-
Inflammation-induced TRPV4 channels exacerbate blood-brain barrier dysfunction in multiple sclerosis.J Neuroinflammation. 2024 Mar 23;21(1):72. doi: 10.1186/s12974-024-03069-9. J Neuroinflammation. 2024. PMID: 38521959 Free PMC article.
-
Transient Receptor Potential Vanilloid 4-Dependent Microglial Function in Myelin Injury and Repair.Int J Mol Sci. 2023 Dec 4;24(23):17097. doi: 10.3390/ijms242317097. Int J Mol Sci. 2023. PMID: 38069420 Free PMC article.
-
Post-ischemic inflammatory response in the brain: Targeting immune cell in ischemic stroke therapy.Front Mol Neurosci. 2023 Apr 3;16:1076016. doi: 10.3389/fnmol.2023.1076016. eCollection 2023. Front Mol Neurosci. 2023. PMID: 37078089 Free PMC article. Review.
-
TRPV4 Channel in Neurological Disease: from Molecular Mechanisms to Therapeutic Potential.Mol Neurobiol. 2025 Mar;62(3):3877-3891. doi: 10.1007/s12035-024-04518-5. Epub 2024 Sep 28. Mol Neurobiol. 2025. PMID: 39333347 Free PMC article. Review.
-
The absence of AQP4/TRPV4 complex substantially reduces acute cytotoxic edema following ischemic injury.Front Cell Neurosci. 2022 Dec 8;16:1054919. doi: 10.3389/fncel.2022.1054919. eCollection 2022. Front Cell Neurosci. 2022. PMID: 36568889 Free PMC article.
References
-
- Arredondo Zamarripa D., Noguez Imm R., Bautista Cortés A. M., Vázquez Ruíz O., Bernardini M., Fiorio Pla A., et al. (2017). Dual contribution of TRPV4 antagonism in the regulatory effect of vasoinhibins on blood-retinal barrier permeability: diabetic milieu makes a difference. Sci. Rep. 7 1–16. 10.1038/s41598-017-13621-8 - DOI - PMC - PubMed
-
- Benfenati V., Caprini M., Dovizio M., Mylonakou M. N., Ferroni S., Ottersen O. P., et al. (2011). An aquaporin-4/transient receptor potential vanilloid 4 (AQP4/TRPV4) complex is essential for cell-volume control in astrocytes. Proc. Natl. Acad. Sci. U.S.A. 108 2563–2568. 10.1073/pnas.1012867108 - DOI - PMC - PubMed
LinkOut - more resources
Full Text Sources
Molecular Biology Databases
Research Materials