BiteOscope, an open platform to study mosquito biting behavior
- PMID: 32960173
- PMCID: PMC7535929
- DOI: 10.7554/eLife.56829
BiteOscope, an open platform to study mosquito biting behavior
Abstract
Female mosquitoes need a blood meal to reproduce, and in obtaining this essential nutrient they transmit deadly pathogens. Although crucial for the spread of mosquito-borne diseases, blood feeding remains poorly understood due to technological limitations. Indeed, studies often expose human subjects to assess biting behavior. Here, we present the biteOscope, a device that attracts mosquitoes to a host mimic which they bite to obtain an artificial blood meal. The host mimic is transparent, allowing high-resolution imaging of the feeding mosquito. Using machine learning, we extract detailed behavioral statistics describing the locomotion, pose, biting, and feeding dynamics of Aedes aegypti, Aedes albopictus, Anopheles stephensi, and Anopheles coluzzii. In addition to characterizing behavioral patterns, we discover that the common insect repellent DEET repels Anopheles coluzzii upon contact with their legs. The biteOscope provides a new perspective on mosquito blood feeding, enabling the high-throughput quantitative characterization of this lethal behavior.
Keywords: Mosquito; behavior; blood feeding; contact-dependent sensing; ecology; ethology; imaging; neuroscience.
Plain language summary
Scientists often sacrifice their own skin to study how mosquitos drink blood. They allow mosquitos to bite them in laboratory settings so they can observe the insects’ feeding behavior. By observing blood feeding, scientists hope to find ways to prevent deadly diseases like malaria, which is transmitted by bites from mosquitos carrying the malaria parasite. These studies are not only unpleasant for the volunteers, they also have important limitations. For example, it is too risky to use pathogen-infected mosquitos that could make the volunteers sick. A device called the biteOscope developed by Hol et al. may give scientists and their skin a reprieve. The device has a transparent skin-like covering that attracts mosquitos and supplies them an artificial blood meal when they bite. The device captures high-resolution images of the insects’ behavior. It is small enough to fit in a backpack when disassembled, costs about $900 to $3,500 US dollars, and is suitable for use in the laboratory or in the field. Using machine-learning techniques, Hol et al. also developed an automated system for analyzing the images. The researchers tested the device on four types of disease-transmitting mosquitos. In one set of experiments, Anopheles mosquitos were recorded interacting with a biteOscope partially coated with an insect repellent called DEET. The images captured by the biteOscope showed that the mosquitos are attracted to the warm surface and land on the part coated with DEET. But when their legs come in contact with the repellent, they leave. The biteOscope provides scientists a new way to study blood feeding, even in mosquitos infected with dangerous pathogens. It might also be used to test new ways to prevent mosquitos from biting and spreading disease. Because the device is portable and relatively inexpensive, it may enable larger studies in a variety of settings.
© 2020, Hol et al.
Conflict of interest statement
FH, LL, MP No competing interests declared
Figures
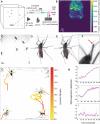
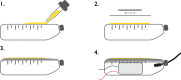
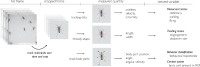
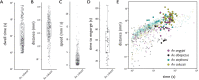
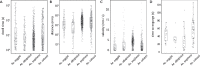
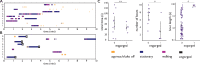
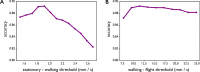
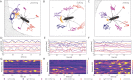
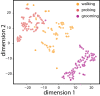
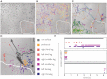
Similar articles
-
Repellent and deterrent effects of SS220, Picaridin, and Deet suppress human blood feeding by Aedes aegypti, Anopheles stephensi, and Phlebotomus papatasi.J Med Entomol. 2006 Jan;43(1):34-9. doi: 10.1603/0022-2585(2006)043[0034:radeos]2.0.co;2. J Med Entomol. 2006. PMID: 16506445
-
Characterizing Mosquito Biting Behavior at High Resolution.Cold Spring Harb Protoc. 2023 Jul 5;2023(7):.pdb.top107658. doi: 10.1101/pdb.top107658. Cold Spring Harb Protoc. 2023. PMID: 36882292 Review.
-
Observing the distribution of mosquito bites on humans to inform personal protection measures against malaria and dengue vectors.PLoS One. 2022 Jul 25;17(7):e0271833. doi: 10.1371/journal.pone.0271833. eCollection 2022. PLoS One. 2022. PMID: 35877666 Free PMC article.
-
Repellent effects on Anopheles arabiensis biting humans in Kruger Park, South Africa.Med Vet Entomol. 2001 Sep;15(3):287-92. doi: 10.1046/j.0269-283x.2001.00309.x. Med Vet Entomol. 2001. PMID: 11583446
-
The efficacy of repellents against Aedes, Anopheles, Culex and Ixodes spp. - a literature review.Travel Med Infect Dis. 2013 Nov-Dec;11(6):374-411. doi: 10.1016/j.tmaid.2013.10.005. Epub 2013 Oct 25. Travel Med Infect Dis. 2013. PMID: 24201040 Review.
Cited by
-
Sustainable Pest Management Using Novel Nanoemulsions of Honeysuckle and Patchouli Essential Oils against the West Nile Virus Vector, Culex pipiens, under Laboratory and Field Conditions.Plants (Basel). 2023 Oct 25;12(21):3682. doi: 10.3390/plants12213682. Plants (Basel). 2023. PMID: 37960039 Free PMC article.
-
Neurogenetic identification of mosquito sensory neurons.iScience. 2023 Apr 18;26(5):106690. doi: 10.1016/j.isci.2023.106690. eCollection 2023 May 19. iScience. 2023. PMID: 37182106 Free PMC article.
-
Behavioral Repertoire on a Vertical Rod-An Ethogram in Dermacentor reticulatus Ticks.Life (Basel). 2022 Dec 13;12(12):2086. doi: 10.3390/life12122086. Life (Basel). 2022. PMID: 36556451 Free PMC article.
-
Automated analysis of feeding behaviors of females of the mosquito Aedes aegypti using a modified flyPAD system.Sci Rep. 2023 Nov 18;13(1):20188. doi: 10.1038/s41598-023-47277-4. Sci Rep. 2023. PMID: 37980438 Free PMC article.
-
A materials-science perspective on tackling COVID-19.Nat Rev Mater. 2020;5(11):847-860. doi: 10.1038/s41578-020-00247-y. Epub 2020 Oct 14. Nat Rev Mater. 2020. PMID: 33078077 Free PMC article. Review.
References
-
- Abboubakar H, Buonomo B, Chitnis N. Modelling the effects of malaria infection on mosquito biting behaviour and attractiveness of humans. Ricerche Di Matematica. 2016;65:329–346. doi: 10.1007/s11587-016-0293-9. - DOI
-
- Allan D, Caswell T, Keim N, van der Wel C. trackpy: Trackpy . v0. 3.2Zenodo. 2016 doi: 10.5281/zenodo.3492186. - DOI
Publication types
MeSH terms
Substances
Grants and funding
LinkOut - more resources
Full Text Sources
Medical