Gene-Editing Technologies Paired With Viral Vectors for Translational Research Into Neurodegenerative Diseases
- PMID: 32903507
- PMCID: PMC7437156
- DOI: 10.3389/fnmol.2020.00148
Gene-Editing Technologies Paired With Viral Vectors for Translational Research Into Neurodegenerative Diseases
Abstract
Diseases of the central nervous system (CNS) have historically been among the most difficult to treat using conventional pharmacological approaches. This is due to a confluence of factors, including the limited regenerative capacity and overall complexity of the brain, problems associated with repeated drug administration, and difficulties delivering drugs across the blood-brain barrier (BBB). Viral-mediated gene transfer represents an attractive alternative for the delivery of therapeutic cargo to the nervous system. Crucially, it usually requires only a single injection, whether that be a gene replacement strategy for an inherited disorder or the delivery of a genome- or epigenome-modifying construct for treatment of CNS diseases and disorders. It is thus understandable that considerable effort has been put towards the development of improved vector systems for gene transfer into the CNS. Different viral vectors are of course tailored to their specific applications, but they generally should share several key properties. The ideal viral vector incorporates a high-packaging capacity, efficient gene transfer paired with robust and sustained expression, lack of oncogenicity, toxicity and pathogenicity, and scalable manufacturing for clinical applications. In this review, we will devote attention to viral vectors derived from human immunodeficiency virus type 1 (lentiviral vectors; LVs) and adeno-associated virus (AAVs). The high interest in these viral delivery systems vectors is due to: (i) robust delivery and long-lasting expression; (ii) efficient transduction into postmitotic cells, including the brain; (iii) low immunogenicity and toxicity; and (iv) compatibility with advanced manufacturing techniques. Here, we will outline basic aspects of LV and AAV biology, particularly focusing on approaches and techniques aiming to enhance viral safety. We will also allocate a significant portion of this review to the development and use of LVs and AAVs for delivery into the CNS, with a focus on the genome and epigenome-editing tools based on clustered regularly interspaced short palindromic repeats/CRISPR-associated protein 9 (CRISPR/Cas 9) and the development of novel strategies for the treatment of neurodegenerative diseases (NDDs).
Keywords: AAV vectors; CRISPR-Cas 9 system; epigenetics (DNA methylation; gene editing; histone modifications); lentiviral (LV) vector; neurodegenarative diseases.
Copyright © 2020 Rittiner, Moncalvo, Chiba-Falek and Kantor.
Figures
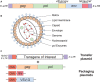
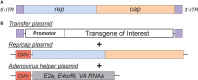
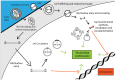
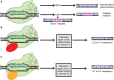
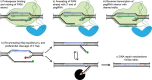
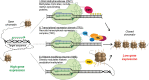
Similar articles
-
Therapeutic modulation of gene expression in the disease state: Treatment strategies and approaches for the development of next-generation of the epigenetic drugs.Front Bioeng Biotechnol. 2022 Oct 17;10:1035543. doi: 10.3389/fbioe.2022.1035543. eCollection 2022. Front Bioeng Biotechnol. 2022. PMID: 36324900 Free PMC article. Review.
-
Lentiviral Vectors for Delivery of Gene-Editing Systems Based on CRISPR/Cas: Current State and Perspectives.Viruses. 2021 Jul 1;13(7):1288. doi: 10.3390/v13071288. Viruses. 2021. PMID: 34372494 Free PMC article. Review.
-
CRISPR Systems Suitable for Single AAV Vector Delivery.Curr Gene Ther. 2022;22(1):1-14. doi: 10.2174/1566523221666211006120355. Curr Gene Ther. 2022. PMID: 34620062 Review.
-
Gene Therapy with CRISPR/Cas9 Coming to Age for HIV Cure.AIDS Rev. 2017 Oct-Dec;19(3):167-172. AIDS Rev. 2017. PMID: 29019352
-
Recent advances in the delivery and applications of nonviral CRISPR/Cas9 gene editing.Drug Deliv Transl Res. 2023 May;13(5):1500-1519. doi: 10.1007/s13346-023-01320-z. Epub 2023 Mar 29. Drug Deliv Transl Res. 2023. PMID: 36988873 Free PMC article. Review.
Cited by
-
Delivery of gene editing therapeutics.Nanomedicine. 2023 Nov;54:102711. doi: 10.1016/j.nano.2023.102711. Epub 2023 Oct 7. Nanomedicine. 2023. PMID: 37813236 Free PMC article. Review.
-
Therapeutic modulation of gene expression in the disease state: Treatment strategies and approaches for the development of next-generation of the epigenetic drugs.Front Bioeng Biotechnol. 2022 Oct 17;10:1035543. doi: 10.3389/fbioe.2022.1035543. eCollection 2022. Front Bioeng Biotechnol. 2022. PMID: 36324900 Free PMC article. Review.
-
Genome editing via non-viral delivery platforms: current progress in personalized cancer therapy.Mol Cancer. 2022 Mar 11;21(1):71. doi: 10.1186/s12943-022-01550-8. Mol Cancer. 2022. PMID: 35277177 Free PMC article. Review.
-
Stem Cell Mimicking Nanoencapsulation for Targeting Arthritis.Int J Nanomedicine. 2021 Dec 31;16:8485-8507. doi: 10.2147/IJN.S334298. eCollection 2021. Int J Nanomedicine. 2021. PMID: 35002240 Free PMC article. Review.
-
Lentiviral mediated delivery of CRISPR/Cas9 reduces intraocular pressure in a mouse model of myocilin glaucoma.Res Sq [Preprint]. 2023 Dec 19:rs.3.rs-3740880. doi: 10.21203/rs.3.rs-3740880/v1. Res Sq. 2023. Update in: Sci Rep. 2024 Mar 23;14(1):6958. doi: 10.1038/s41598-024-57286-6 PMID: 38196579 Free PMC article. Updated. Preprint.
References
Grants and funding
LinkOut - more resources
Full Text Sources