TRIBE editing reveals specific mRNA targets of eIF4E-BP in Drosophila and in mammals
- PMID: 32851185
- PMCID: PMC7423359
- DOI: 10.1126/sciadv.abb8771
TRIBE editing reveals specific mRNA targets of eIF4E-BP in Drosophila and in mammals
Abstract
4E-BP (eIF4E-BP) represses translation initiation by binding to the 5' cap-binding protein eIF4E and inhibiting its activity. Although 4E-BP has been shown to be important in growth control, stress response, cancer, neuronal activity, and mammalian circadian rhythms, it is not understood how it preferentially represses a subset of mRNAs. We successfully used HyperTRIBE (targets of RNA binding proteins identified by editing) to identify in vivo 4E-BP mRNA targets in both Drosophila and mammals under conditions known to activate 4E-BP. The protein associates with specific mRNAs, and ribosome profiling data show that mTOR inhibition changes the translational efficiency of 4E-BP TRIBE targets more substantially compared to nontargets. In both systems, these targets have specific motifs and are enriched in translation-related pathways, which correlate well with the known activity of 4E-BP and suggest that it modulates the binding specificity of eIF4E and contributes to mTOR translational specificity.
Copyright © 2020 The Authors, some rights reserved; exclusive licensee American Association for the Advancement of Science. No claim to original U.S. Government Works. Distributed under a Creative Commons Attribution NonCommercial License 4.0 (CC BY-NC).
Figures
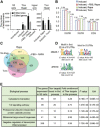
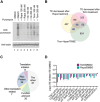
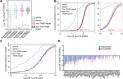
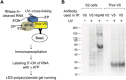
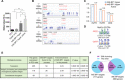
Similar articles
-
The 4E-BP Caf20p Mediates Both eIF4E-Dependent and Independent Repression of Translation.PLoS Genet. 2015 May 14;11(5):e1005233. doi: 10.1371/journal.pgen.1005233. eCollection 2015 May. PLoS Genet. 2015. PMID: 25973932 Free PMC article.
-
Inhibition of Mitogen-activated Protein Kinase (MAPK)-interacting Kinase (MNK) Preferentially Affects Translation of mRNAs Containing Both a 5'-Terminal Cap and Hairpin.J Biol Chem. 2016 Feb 12;291(7):3455-67. doi: 10.1074/jbc.M115.694190. Epub 2015 Dec 14. J Biol Chem. 2016. PMID: 26668315 Free PMC article.
-
A unifying model for mTORC1-mediated regulation of mRNA translation.Nature. 2012 May 2;485(7396):109-13. doi: 10.1038/nature11083. Nature. 2012. PMID: 22552098 Free PMC article.
-
The mTOR/4E-BP1/eIF4E Signalling Pathway as a Source of Cancer Drug Targets.Curr Med Chem. 2022;29(20):3501-3529. doi: 10.2174/0929867329666220224112042. Curr Med Chem. 2022. PMID: 35209811 Review.
-
Dual targeting of eIF4E by blocking MNK and mTOR pathways in leukemia.Cytokine. 2017 Jan;89:116-121. doi: 10.1016/j.cyto.2016.01.024. Epub 2016 Apr 16. Cytokine. 2017. PMID: 27094611 Free PMC article. Review.
Cited by
-
DDX3 depletion represses translation of mRNAs with complex 5' UTRs.Nucleic Acids Res. 2021 May 21;49(9):5336-5350. doi: 10.1093/nar/gkab287. Nucleic Acids Res. 2021. PMID: 33905506 Free PMC article.
-
Antagonistic roles for Ataxin-2 structured and disordered domains in RNP condensation.Elife. 2021 Mar 10;10:e60326. doi: 10.7554/eLife.60326. Elife. 2021. PMID: 33689682 Free PMC article.
-
Distinct roles of LARP1 and 4EBP1/2 in regulating translation and stability of 5'TOP mRNAs.Sci Adv. 2024 Feb 16;10(7):eadi7830. doi: 10.1126/sciadv.adi7830. Epub 2024 Feb 16. Sci Adv. 2024. PMID: 38363833 Free PMC article.
-
Comparison of TRIBE and STAMP for identifying targets of RNA binding proteins in human and Drosophila cells.RNA. 2023 Aug;29(8):1230-1242. doi: 10.1261/rna.079608.123. Epub 2023 May 11. RNA. 2023. PMID: 37169395 Free PMC article.
-
Abundance of Amino Acid Transporters and mTOR Pathway Components in the Gastrointestinal Tract of Lactating Holstein Cows.Animals (Basel). 2023 Mar 29;13(7):1189. doi: 10.3390/ani13071189. Animals (Basel). 2023. PMID: 37048445 Free PMC article.
References
-
- Mamane Y., Petroulakis E., LeBacquer O., Sonenberg N., mTOR, translation initiation and cancer. Oncogene 25, 6416–6422 (2006). - PubMed
Publication types
MeSH terms
Substances
Grants and funding
LinkOut - more resources
Full Text Sources
Medical
Molecular Biology Databases
Miscellaneous