Emerging importance of satellite glia in nervous system function and dysfunction
- PMID: 32699292
- PMCID: PMC7374656
- DOI: 10.1038/s41583-020-0333-z
Emerging importance of satellite glia in nervous system function and dysfunction
Erratum in
-
Author Correction: Emerging importance of satellite glia in nervous system function and dysfunction.Nat Rev Neurosci. 2020 Dec;21(12):732. doi: 10.1038/s41583-020-00402-y. Nat Rev Neurosci. 2020. PMID: 33093636 Free PMC article.
Abstract
Satellite glial cells (SGCs) closely envelop cell bodies of neurons in sensory, sympathetic and parasympathetic ganglia. This unique organization is not found elsewhere in the nervous system. SGCs in sensory ganglia are activated by numerous types of nerve injury and inflammation. The activation includes upregulation of glial fibrillary acidic protein, stronger gap junction-mediated SGC-SGC and neuron-SGC coupling, increased sensitivity to ATP, downregulation of Kir4.1 potassium channels and increased cytokine synthesis and release. There is evidence that these changes in SGCs contribute to chronic pain by augmenting neuronal activity and that these changes are consistent in various rodent pain models and likely also in human pain. Therefore, understanding these changes and the resulting abnormal interactions of SGCs with sensory neurons could provide a mechanistic approach that might be exploited therapeutically in alleviation and prevention of pain. We describe how SGCs are altered in rodent models of four common types of pain: systemic inflammation (sickness behaviour), post-surgical pain, diabetic neuropathic pain and post-herpetic pain.
Conflict of interest statement
The authors declare no competing interests.
Figures
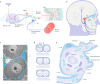
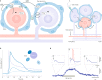
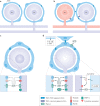
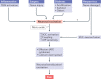
Similar articles
-
Gliopathic pain: when satellite glial cells go bad.Neuroscientist. 2009 Oct;15(5):450-63. doi: 10.1177/1073858409336094. Neuroscientist. 2009. PMID: 19826169 Free PMC article. Review.
-
The effects of sympathetic nerve damage on satellite glial cells in the mouse superior cervical ganglion.Auton Neurosci. 2019 Nov;221:102584. doi: 10.1016/j.autneu.2019.102584. Epub 2019 Aug 24. Auton Neurosci. 2019. PMID: 31494528
-
Intercellular communication in sensory ganglia by purinergic receptors and gap junctions: implications for chronic pain.Brain Res. 2012 Dec 3;1487:183-91. doi: 10.1016/j.brainres.2012.03.070. Epub 2012 Jul 4. Brain Res. 2012. PMID: 22771859 Review.
-
Changes in the expression of satellite glial cell-specific markers during postnatal development of rat sympathetic ganglia.Brain Res. 2024 Apr 15;1829:148809. doi: 10.1016/j.brainres.2024.148809. Epub 2024 Feb 12. Brain Res. 2024. PMID: 38354998
-
How Is Peripheral Injury Signaled to Satellite Glial Cells in Sensory Ganglia?Cells. 2022 Feb 1;11(3):512. doi: 10.3390/cells11030512. Cells. 2022. PMID: 35159321 Free PMC article. Review.
Cited by
-
Modulation of Glia Activation by TRPA1 Antagonism in Preclinical Models of Migraine.Int J Mol Sci. 2022 Nov 15;23(22):14085. doi: 10.3390/ijms232214085. Int J Mol Sci. 2022. PMID: 36430567 Free PMC article.
-
Glial cell alterations in diabetes-induced neurodegeneration.Cell Mol Life Sci. 2024 Jan 18;81(1):47. doi: 10.1007/s00018-023-05024-y. Cell Mol Life Sci. 2024. PMID: 38236305 Free PMC article. Review.
-
Trigeminal ganglion itself can be a viable target to manage trigeminal neuralgia.J Headache Pain. 2022 Nov 24;23(1):150. doi: 10.1186/s10194-022-01512-x. J Headache Pain. 2022. PMID: 36424545 Free PMC article. Review.
-
Satellite glia modulate sympathetic neuron survival, activity, and autonomic function.Elife. 2022 Aug 23;11:e74295. doi: 10.7554/eLife.74295. Elife. 2022. PMID: 35997251 Free PMC article.
-
Dangerous Behavior and Intractable Axial Skeletal Pain in Performance Horses: A Possible Role for Ganglioneuritis (14 Cases; 2014-2019).Front Vet Sci. 2021 Dec 10;8:734218. doi: 10.3389/fvets.2021.734218. eCollection 2021. Front Vet Sci. 2021. PMID: 34957274 Free PMC article.
References
Publication types
MeSH terms
Grants and funding
LinkOut - more resources
Full Text Sources
Other Literature Sources
Medical
Miscellaneous