Half a century of amyloids: past, present and future
- PMID: 32632432
- PMCID: PMC7445747
- DOI: 10.1039/c9cs00199a
Half a century of amyloids: past, present and future
Abstract
Amyloid diseases are global epidemics with profound health, social and economic implications and yet remain without a cure. This dire situation calls for research into the origin and pathological manifestations of amyloidosis to stimulate continued development of new therapeutics. In basic science and engineering, the cross-β architecture has been a constant thread underlying the structural characteristics of pathological and functional amyloids, and realizing that amyloid structures can be both pathological and functional in nature has fuelled innovations in artificial amyloids, whose use today ranges from water purification to 3D printing. At the conclusion of a half century since Eanes and Glenner's seminal study of amyloids in humans, this review commemorates the occasion by documenting the major milestones in amyloid research to date, from the perspectives of structural biology, biophysics, medicine, microbiology, engineering and nanotechnology. We also discuss new challenges and opportunities to drive this interdisciplinary field moving forward.
Conflict of interest statement
Conflicts of interest
DSE is SAB chair and equity holder in ADDRx, Inc.
Figures
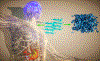
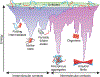
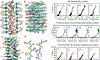
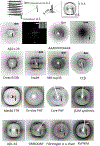
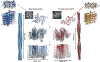
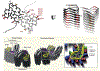
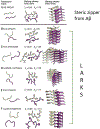
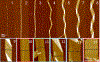
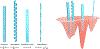
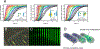
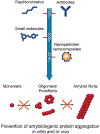
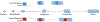
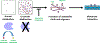
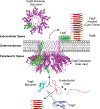
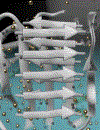
Similar articles
-
Depressing time: Waiting, melancholia, and the psychoanalytic practice of care.In: Kirtsoglou E, Simpson B, editors. The Time of Anthropology: Studies of Contemporary Chronopolitics. Abingdon: Routledge; 2020. Chapter 5. In: Kirtsoglou E, Simpson B, editors. The Time of Anthropology: Studies of Contemporary Chronopolitics. Abingdon: Routledge; 2020. Chapter 5. PMID: 36137063 Free Books & Documents. Review.
-
Unlocking data: Decision-maker perspectives on cross-sectoral data sharing and linkage as part of a whole-systems approach to public health policy and practice.Public Health Res (Southampt). 2024 Nov 20:1-30. doi: 10.3310/KYTW2173. Online ahead of print. Public Health Res (Southampt). 2024. PMID: 39582242
-
Dynamic Field Theory of Executive Function: Identifying Early Neurocognitive Markers.Monogr Soc Res Child Dev. 2024 Dec;89(3):7-109. doi: 10.1111/mono.12478. Monogr Soc Res Child Dev. 2024. PMID: 39628288 Free PMC article.
-
Place-Based Developmental Research: Conceptual and Methodological Advances in Studying Youth Development in Context.Monogr Soc Res Child Dev. 2023 Dec;88(3):7-130. doi: 10.1111/mono.12472. Monogr Soc Res Child Dev. 2023. PMID: 37953661 Free PMC article.
-
Trends in Surgical and Nonsurgical Aesthetic Procedures: A 14-Year Analysis of the International Society of Aesthetic Plastic Surgery-ISAPS.Aesthetic Plast Surg. 2024 Oct;48(20):4217-4227. doi: 10.1007/s00266-024-04260-2. Epub 2024 Aug 5. Aesthetic Plast Surg. 2024. PMID: 39103642 Review.
Cited by
-
Catalytically Active Amyloids as Future Bionanomaterials.Nanomaterials (Basel). 2022 Oct 28;12(21):3802. doi: 10.3390/nano12213802. Nanomaterials (Basel). 2022. PMID: 36364578 Free PMC article. Review.
-
Staphylococcus aureus functional amyloids catalyze degradation of β-lactam antibiotics.Nat Commun. 2023 Dec 11;14(1):8198. doi: 10.1038/s41467-023-43624-1. Nat Commun. 2023. PMID: 38081813 Free PMC article.
-
A central role for amyloid fibrin microclots in long COVID/PASC: origins and therapeutic implications.Biochem J. 2022 Feb 17;479(4):537-559. doi: 10.1042/BCJ20220016. Biochem J. 2022. PMID: 35195253 Free PMC article. Review.
-
Local Insulin-Derived Amyloidosis Model Confronted with Silymarin: Histological Insights and Gene Expression of MMP, TNF-α, and IL-6.Int J Mol Sci. 2022 Apr 29;23(9):4952. doi: 10.3390/ijms23094952. Int J Mol Sci. 2022. PMID: 35563343 Free PMC article.
-
Oat Plant Amyloids for Sustainable Functional Materials.Adv Sci (Weinh). 2022 Feb;9(4):e2104445. doi: 10.1002/advs.202104445. Epub 2021 Dec 20. Adv Sci (Weinh). 2022. PMID: 34931493 Free PMC article.
References
Publication types
MeSH terms
Substances
Grants and funding
LinkOut - more resources
Full Text Sources
Medical
Miscellaneous