Cas9-AAV6-engineered human mesenchymal stromal cells improved cutaneous wound healing in diabetic mice
- PMID: 32424320
- PMCID: PMC7235221
- DOI: 10.1038/s41467-020-16065-3
Cas9-AAV6-engineered human mesenchymal stromal cells improved cutaneous wound healing in diabetic mice
Abstract
Human mesenchymal stromal cells (hMSCs) are a promising source for engineered cell-based therapies in which genetic engineering could enhance therapeutic efficacy and install novel cellular functions. Here, we describe an optimized Cas9-AAV6-based genome editing tool platform for site-specific mutagenesis and integration of up to more than 3 kilobases of exogenous DNA in the genome of hMSCs derived from the bone marrow, adipose tissue, and umbilical cord blood without altering their ex vivo characteristics. We generate safe harbor-integrated lines of engineered hMSCs and show that engineered luciferase-expressing hMSCs are transiently active in vivo in wound beds of db/db mice. Moreover, we generate PDGF-BB- and VEGFA-hypersecreting hMSC lines as short-term, local wound healing agents with superior therapeutic efficacy over wildtype hMSCs in the diabetic mouse model without replacing resident cells long-term. This study establishes a precise genetic engineering platform for genetic studies of hMSCs and development of engineered hMSC-based therapies.
Conflict of interest statement
M.P. serves on the SAB and has equity in CRISPR Tx and Allogene Tx. Neither company had input into the work described in the manuscript. All other authors declare no competing interests.
Figures

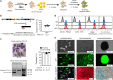
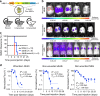
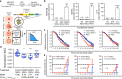
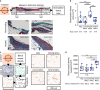
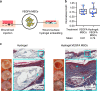
Similar articles
-
Neurotrophin-3 accelerates wound healing in diabetic mice by promoting a paracrine response in mesenchymal stem cells.Cell Transplant. 2013;22(6):1011-21. doi: 10.3727/096368912X657495. Epub 2012 Oct 3. Cell Transplant. 2013. PMID: 23043768
-
Pooled human bone marrow-derived mesenchymal stromal cells with defined trophic factors cargo promote dermal wound healing in diabetic rats by improved vascularization and dynamic recruitment of M2-like macrophages.Front Immunol. 2022 Aug 19;13:976511. doi: 10.3389/fimmu.2022.976511. eCollection 2022. Front Immunol. 2022. PMID: 36059533 Free PMC article.
-
Platelet-derived growth factor BB mediates the tropism of human mesenchymal stem cells for malignant gliomas.Neurosurgery. 2010 Jan;66(1):144-56; discussion 156-7. doi: 10.1227/01.NEU.0000363149.58885.2E. Neurosurgery. 2010. PMID: 20023545 Free PMC article.
-
Adipose-derived mesenchymal stem cells accelerate diabetic wound healing in a similar fashion as bone marrow-derived cells.Am J Physiol Cell Physiol. 2018 Dec 1;315(6):C885-C896. doi: 10.1152/ajpcell.00120.2018. Epub 2018 Nov 7. Am J Physiol Cell Physiol. 2018. PMID: 30404559 Free PMC article.
-
IL-7 overexpression enhances therapeutic potential of rat bone marrow mesenchymal stem cells for diabetic wounds.Wound Repair Regen. 2019 May;27(3):235-248. doi: 10.1111/wrr.12706. Epub 2019 Mar 6. Wound Repair Regen. 2019. PMID: 30761686
Cited by
-
Heterogeneity of In Vitro Expanded Mesenchymal Stromal Cells and Strategies to Improve Their Therapeutic Actions.Pharmaceutics. 2022 May 23;14(5):1112. doi: 10.3390/pharmaceutics14051112. Pharmaceutics. 2022. PMID: 35631698 Free PMC article. Review.
-
Innovative Cell and Platelet Rich Plasma Therapies for Diabetic Foot Ulcer Treatment: The Allogeneic Approach.Front Bioeng Biotechnol. 2022 May 2;10:869408. doi: 10.3389/fbioe.2022.869408. eCollection 2022. Front Bioeng Biotechnol. 2022. PMID: 35586557 Free PMC article. Review.
-
Extracellular vesicles modulate key signalling pathways in refractory wound healing.Burns Trauma. 2023 Nov 16;11:tkad039. doi: 10.1093/burnst/tkad039. eCollection 2023. Burns Trauma. 2023. PMID: 38026441 Free PMC article. Review.
-
Scaffold-based delivery of mesenchymal stromal cells to diabetic wounds.Stem Cell Res Ther. 2022 Aug 20;13(1):426. doi: 10.1186/s13287-022-03115-4. Stem Cell Res Ther. 2022. PMID: 35987712 Free PMC article. Review.
-
Stem Cell Mimicking Nanoencapsulation for Targeting Arthritis.Int J Nanomedicine. 2021 Dec 31;16:8485-8507. doi: 10.2147/IJN.S334298. eCollection 2021. Int J Nanomedicine. 2021. PMID: 35002240 Free PMC article. Review.
References
Publication types
MeSH terms
Substances
Grants and funding
LinkOut - more resources
Full Text Sources
Miscellaneous