AMPAR Auxiliary Protein SHISA6 Facilitates Purkinje Cell Synaptic Excitability and Procedural Memory Formation
- PMID: 32294428
- PMCID: PMC7175376
- DOI: 10.1016/j.celrep.2020.03.079
AMPAR Auxiliary Protein SHISA6 Facilitates Purkinje Cell Synaptic Excitability and Procedural Memory Formation
Abstract
The majority of excitatory postsynaptic currents in the brain are gated through AMPA-type glutamate receptors, the kinetics and trafficking of which can be modulated by auxiliary proteins. It remains to be elucidated whether and how auxiliary proteins can modulate synaptic function to contribute to procedural memory formation. In this study, we report that the AMPA-type glutamate receptor (AMPAR) auxiliary protein SHISA6 (CKAMP52) is expressed in cerebellar Purkinje cells, where it co-localizes with GluA2-containing AMPARs. The absence of SHISA6 in Purkinje cells results in severe impairments in the adaptation of the vestibulo-ocular reflex and eyeblink conditioning. The physiological abnormalities include decreased presence of AMPARs in synaptosomes, impaired excitatory transmission, increased deactivation of AMPA receptors, and reduced induction of long-term potentiation at Purkinje cell synapses. Our data indicate that Purkinje cells require SHISA6-dependent modification of AMPAR function in order to facilitate cerebellar, procedural memory formation.
Keywords: AMPAR kinetics; auxiliary subunit; cerebellum; long-term depression; procedural memory; synapse.
Copyright © 2020 The Author(s). Published by Elsevier Inc. All rights reserved.
Conflict of interest statement
Declaration of Interests A.B.S. participates in a holding that owns shares of Sylics BV.
Figures
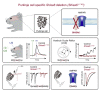
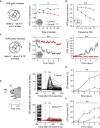
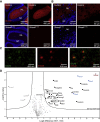
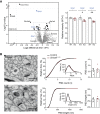
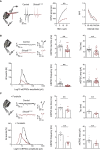
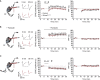
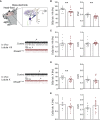
Similar articles
-
Shisa6 traps AMPA receptors at postsynaptic sites and prevents their desensitization during synaptic activity.Nat Commun. 2016 Mar 2;7:10682. doi: 10.1038/ncomms10682. Nat Commun. 2016. PMID: 26931375 Free PMC article.
-
Motor Learning Requires Purkinje Cell Synaptic Potentiation through Activation of AMPA-Receptor Subunit GluA3.Neuron. 2017 Jan 18;93(2):409-424. doi: 10.1016/j.neuron.2016.11.046. Neuron. 2017. PMID: 28103481 Free PMC article.
-
The AMPA receptor-associated protein Shisa7 regulates hippocampal synaptic function and contextual memory.Elife. 2017 Dec 4;6:e24192. doi: 10.7554/eLife.24192. Elife. 2017. PMID: 29199957 Free PMC article.
-
Modulation of information processing by AMPA receptor auxiliary subunits.J Physiol. 2021 Jan;599(2):471-483. doi: 10.1113/JP276698. Epub 2020 Jul 24. J Physiol. 2021. PMID: 32628275 Review.
-
Structural and Functional Architecture of AMPA-Type Glutamate Receptors and Their Auxiliary Proteins.Neuron. 2017 May 17;94(4):713-730. doi: 10.1016/j.neuron.2017.04.009. Neuron. 2017. PMID: 28521126 Review.
Cited by
-
Chemically defined and small molecules-based generation of sinoatrial node-like cells.Stem Cell Res Ther. 2022 Apr 11;13(1):158. doi: 10.1186/s13287-022-02834-y. Stem Cell Res Ther. 2022. PMID: 35410454 Free PMC article.
-
Transcranial direct current stimulation of cerebellum alters spiking precision in cerebellar cortex: A modeling study of cellular responses.PLoS Comput Biol. 2021 Dec 9;17(12):e1009609. doi: 10.1371/journal.pcbi.1009609. eCollection 2021 Dec. PLoS Comput Biol. 2021. PMID: 34882680 Free PMC article.
-
Neonatal Sevoflurane Exposure Impairs Learning and Memory by the Hypermethylation of Hippocampal Synaptic Genes.Mol Neurobiol. 2021 Mar;58(3):895-904. doi: 10.1007/s12035-020-02161-4. Epub 2020 Oct 14. Mol Neurobiol. 2021. PMID: 33052583
-
Auxiliary Subunits Regulate the Dendritic Turnover of AMPA Receptors in Mouse Hippocampal Neurons.Front Mol Neurosci. 2021 Aug 23;14:728498. doi: 10.3389/fnmol.2021.728498. eCollection 2021. Front Mol Neurosci. 2021. PMID: 34497491 Free PMC article.
-
Shisa6 mediates cell-type specific regulation of depression in the nucleus accumbens.Mol Psychiatry. 2021 Dec;26(12):7316-7327. doi: 10.1038/s41380-021-01217-8. Epub 2021 Jul 12. Mol Psychiatry. 2021. PMID: 34253865 Free PMC article.
References
-
- Barski J.J., Dethleffsen K., Meyer M. Cre recombinase expression in cerebellar Purkinje cells. Genesis. 2000;28:93–98. - PubMed
-
- Bates D., Mächler M., Bolker B., Walker S. Fitting linear mixed-effects models using lme4. J. Stat. Softw. 2015;67
-
- Bruderer R., Bernhardt O.M., Gandhi T., Miladinović S.M., Cheng L.Y., Messner S., Ehrenberger T., Zanotelli V., Butscheid Y., Escher C. Extending the limits of quantitative proteome profiling with data-independent acquisition and application to acetaminophen-treated three-dimensional liver microtissues. Mol. Cell. Proteomics. 2015;14:1400–1410. - PMC - PubMed
-
- Chen L., Chetkovich D.M., Petralia R.S., Sweeney N.T., Kawasaki Y., Wenthold R.J., Bredt D.S., Nicoll R.A. Stargazin regulates synaptic targeting of AMPA receptors by two distinct mechanisms. Nature. 2000;408:936–943. - PubMed
-
- Chen N., Pandya N.J., Koopmans F., Castelo-Székelv V., van der Schors R.C., Smit A.B., Li K.W. Interaction proteomics reveals brain region-specific AMPA receptor complexes. J. Proteome Res. 2014;13:5695–5706. - PubMed
Publication types
MeSH terms
Substances
LinkOut - more resources
Full Text Sources
Molecular Biology Databases