Structure and function of the calcium-selective TRP channel TRPV6
- PMID: 32073143
- PMCID: PMC7689878
- DOI: 10.1113/JP279024
Structure and function of the calcium-selective TRP channel TRPV6
Abstract
Epithelial calcium channel TRPV6 is a member of the vanilloid subfamily of TRP channels that is permeable to cations and highly selective to Ca2+ ; it shows constitutive activity regulated negatively by Ca2+ and positively by phosphoinositol and cholesterol lipids. In this review, we describe the molecular structure of TRPV6 and discuss how its structural elements define its unique functional properties. High Ca2+ selectivity of TRPV6 originates from the narrow selectivity filter, where Ca2+ ions are directly coordinated by a ring of anionic aspartate side chains. Divalent cations Ca2+ and Ba2+ permeate TRPV6 pore according to the knock-off mechanism, while tight binding of Gd3+ to the aspartate ring blocks the channel and prevents Na+ from permeating the pore. The iris-like channel opening is accompanied by an α-to-π helical transition in the pore-lining transmembrane helix S6. As a result of this transition, the intracellular halves of the S6 helices bend and rotate by about 100 deg, exposing different residues to the channel pore in the open and closed states. Channel opening is also associated with changes in occupancy of the transmembrane domain lipid binding sites. The inhibitor 2-aminoethoxydiphenyl borate (2-APB) binds to TRPV6 in a pocket formed by the cytoplasmic half of the S1-S4 transmembrane helical bundle and shifts open-closed channel equilibrium towards the closed state by outcompeting lipids critical for activation. Ca2+ inhibits TRPV6 via binding to calmodulin (CaM), which mediates Ca2+ -dependent inactivation. The TRPV6-CaM complex exhibits 1:1 stoichiometry; one TRPV6 tetramer binds both CaM lobes, which adopt a distinct head-to-tail arrangement. The CaM C-terminal lobe plugs the channel through a unique cation-π interaction by inserting the side chain of lysine K115 into a tetra-tryptophan cage at the ion channel pore intracellular entrance. Recent studies of TRPV6 structure and function described in this review advance our understanding of the role of this channel in physiology and pathophysiology and inform new therapeutic design.
Keywords: TRP channels; X-ray crystallography; calcium; cryo-EM; gating; ion channels; lipids; structure.
© 2020 The Authors. The Journal of Physiology © 2020 The Physiological Society.
Conflict of interest statement
Additional information
Competing interests
The authors declare no competing interests.
Figures
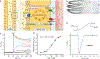
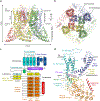
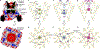
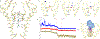
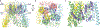
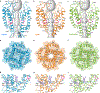
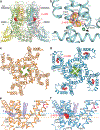
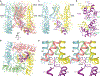
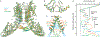
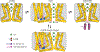
Similar articles
-
Mechanism of calmodulin inactivation of the calcium-selective TRP channel TRPV6.Sci Adv. 2018 Aug 15;4(8):eaau6088. doi: 10.1126/sciadv.aau6088. eCollection 2018 Aug. Sci Adv. 2018. PMID: 30116787 Free PMC article.
-
Opening of the human epithelial calcium channel TRPV6.Nature. 2018 Jan 11;553(7687):233-237. doi: 10.1038/nature25182. Epub 2017 Dec 20. Nature. 2018. PMID: 29258289 Free PMC article.
-
Determining the Crystal Structure of TRPV6.In: Kozak JA, Putney JW Jr, editors. Calcium Entry Channels in Non-Excitable Cells. Boca Raton (FL): CRC Press/Taylor & Francis; 2018. Chapter 14. In: Kozak JA, Putney JW Jr, editors. Calcium Entry Channels in Non-Excitable Cells. Boca Raton (FL): CRC Press/Taylor & Francis; 2018. Chapter 14. PMID: 30299652 Free Books & Documents. Review.
-
Structural bases of TRP channel TRPV6 allosteric modulation by 2-APB.Nat Commun. 2018 Jun 25;9(1):2465. doi: 10.1038/s41467-018-04828-y. Nat Commun. 2018. PMID: 29941865 Free PMC article.
-
What structures did, and did not, reveal about the function of the epithelial Ca2+ channels TRPV5 and TRPV6.Cell Calcium. 2022 Sep;106:102620. doi: 10.1016/j.ceca.2022.102620. Epub 2022 Jul 3. Cell Calcium. 2022. PMID: 35834842 Free PMC article. Review.
Cited by
-
Recent Developments on the Roles of Calcium Signals and Potential Therapy Targets in Cervical Cancer.Cells. 2022 Sep 26;11(19):3003. doi: 10.3390/cells11193003. Cells. 2022. PMID: 36230965 Free PMC article. Review.
-
Bidirectional Allosteric Coupling between PIP2 Binding and the Pore of the Oncochannel TRPV6.Int J Mol Sci. 2024 Jan 3;25(1):618. doi: 10.3390/ijms25010618. Int J Mol Sci. 2024. PMID: 38203789 Free PMC article.
-
P-Loop Channels: Experimental Structures, and Physics-Based and Neural Networks-Based Models.Membranes (Basel). 2022 Feb 16;12(2):229. doi: 10.3390/membranes12020229. Membranes (Basel). 2022. PMID: 35207150 Free PMC article. Review.
-
Structural mechanisms of TRPV6 inhibition by ruthenium red and econazole.Nat Commun. 2021 Nov 1;12(1):6284. doi: 10.1038/s41467-021-26608-x. Nat Commun. 2021. PMID: 34725357 Free PMC article.
-
The TRPV6 Calcium Channel and Its Relationship with Cancer.Biology (Basel). 2024 Mar 5;13(3):168. doi: 10.3390/biology13030168. Biology (Basel). 2024. PMID: 38534438 Free PMC article. Review.
References
-
- Barbato G, Ikura M, Kay LE, Pastor RW, and Bax A (1992). Backbone dynamics of calmodulin studied by 15N relaxation using inverse detected two-dimensional NMR spectroscopy: the central helix is flexible. Biochemistry 31, 5269–5278. - PubMed
-
- Bate N, Caves RE, Skinner SP, Goult BT, Basran J, Mitcheson JS, and Vuister GW (2018). A Novel Mechanism for Calmodulin-Dependent Inactivation of Transient Receptor Potential Vanilloid 6. Biochemistry 57, 2611–2622. - PubMed
Publication types
MeSH terms
Substances
Grants and funding
- R01 CA206573/GF/NIH HHS/United States
- R01 NS083660/GF/NIH HHS/United States
- 1818213/CD/ODCDC CDC HHS/United States
- R01 CA206573/CA/NCI NIH HHS/United States
- CA206573/CA/NCI NIH HHS/United States
- R01 NS107253/NS/NINDS NIH HHS/United States
- NS107253/NS/NINDS NIH HHS/United States
- R01 NS083660/NS/NINDS NIH HHS/United States
- R01 GM128195/GF/NIH HHS/United States
- R01 NS107253/GF/NIH HHS/United States
- R01 GM128195/GM/NIGMS NIH HHS/United States
- 1818213/CD/ODCDC CDC HHS/United States
- NS107253/NS/NINDS NIH HHS/United States
- CA206573/CA/NCI NIH HHS/United States
LinkOut - more resources
Full Text Sources
Research Materials
Miscellaneous