Structural mechanisms of transient receptor potential ion channels
- PMID: 31972006
- PMCID: PMC7054860
- DOI: 10.1085/jgp.201811998
Structural mechanisms of transient receptor potential ion channels
Abstract
Transient receptor potential (TRP) ion channels are evolutionarily ancient sensory proteins that detect and integrate a wide range of physical and chemical stimuli. TRP channels are fundamental for numerous biological processes and are therefore associated with a multitude of inherited and acquired human disorders. In contrast to many other major ion channel families, high-resolution structures of TRP channels were not available before 2013. Remarkably, however, the subsequent "resolution revolution" in cryo-EM has led to an explosion of TRP structures in the last few years. These structures have confirmed that TRP channels assemble as tetramers and resemble voltage-gated ion channels in their overall architecture. But beyond the relatively conserved transmembrane core embedded within the lipid bilayer, each TRP subtype appears to be endowed with a unique set of soluble domains that may confer diverse regulatory mechanisms. Importantly, TRP channel TR structures have revealed sites and mechanisms of action of numerous synthetic and natural compounds, as well as those for endogenous ligands such as lipids, Ca2+, and calmodulin. Here, I discuss these recent findings with a particular focus on the conserved transmembrane region and how these structures may help to rationally target this important class of ion channels for the treatment of numerous human conditions.
© 2020 Cao.
Figures
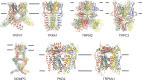
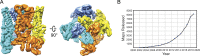
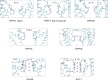
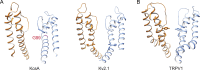
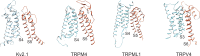
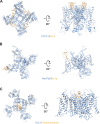
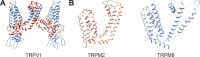
Similar articles
-
Structural and Evolutionary Insights Point to Allosteric Regulation of TRP Ion Channels.Acc Chem Res. 2019 Jun 18;52(6):1643-1652. doi: 10.1021/acs.accounts.9b00075. Epub 2019 May 31. Acc Chem Res. 2019. PMID: 31149807 Free PMC article.
-
Determining the Crystal Structure of TRPV6.In: Kozak JA, Putney JW Jr, editors. Calcium Entry Channels in Non-Excitable Cells. Boca Raton (FL): CRC Press/Taylor & Francis; 2018. Chapter 14. In: Kozak JA, Putney JW Jr, editors. Calcium Entry Channels in Non-Excitable Cells. Boca Raton (FL): CRC Press/Taylor & Francis; 2018. Chapter 14. PMID: 30299652 Free Books & Documents. Review.
-
Structural insights into the gating mechanisms of TRPV channels.Cell Calcium. 2020 May;87:102168. doi: 10.1016/j.ceca.2020.102168. Epub 2020 Jan 24. Cell Calcium. 2020. PMID: 32004816 Free PMC article. Review.
-
Structural Pharmacology of TRP Channels.J Mol Biol. 2021 Aug 20;433(17):166914. doi: 10.1016/j.jmb.2021.166914. Epub 2021 Mar 5. J Mol Biol. 2021. PMID: 33676926 Free PMC article. Review.
-
Recent progress in structural studies on canonical TRP ion channels.Cell Calcium. 2019 Nov;83:102075. doi: 10.1016/j.ceca.2019.102075. Epub 2019 Aug 28. Cell Calcium. 2019. PMID: 31491644 Review.
Cited by
-
Genetically-encoded BRET probes shed light on ligand bias-induced variable ion selectivity in TRPV1 and P2X5/7.Proc Natl Acad Sci U S A. 2022 Nov 16;119(46):e2205207119. doi: 10.1073/pnas.2205207119. Epub 2022 Nov 7. Proc Natl Acad Sci U S A. 2022. PMID: 36343259 Free PMC article.
-
Therapeutic potential of transient receptor potential (TRP) channels in psychiatric disorders.J Neural Transm (Vienna). 2024 Sep;131(9):1025-1037. doi: 10.1007/s00702-024-02803-0. Epub 2024 Jul 15. J Neural Transm (Vienna). 2024. PMID: 39007920 Review.
-
Spinal Reflex Control of Arterial Blood Pressure: The Role of TRP Channels and Their Endogenous Eicosanoid Modulators.Front Physiol. 2022 Feb 23;13:838175. doi: 10.3389/fphys.2022.838175. eCollection 2022. Front Physiol. 2022. PMID: 35283783 Free PMC article. Review.
-
Hunting with heat: thermosensory-driven foraging in mosquitoes, snakes and beetles.J Exp Biol. 2023 Jul 1;226(13):jeb229658. doi: 10.1242/jeb.229658. Epub 2023 Jun 29. J Exp Biol. 2023. PMID: 37382467 Free PMC article.
-
TRPM3 in Brain (Patho)Physiology.Front Cell Dev Biol. 2021 Feb 26;9:635659. doi: 10.3389/fcell.2021.635659. eCollection 2021. Front Cell Dev Biol. 2021. PMID: 33732703 Free PMC article. Review.
References
Publication types
MeSH terms
Substances
Grants and funding
LinkOut - more resources
Full Text Sources
Miscellaneous