Prox1-positive cells monitor and sustain the murine intestinal epithelial cholinergic niche
- PMID: 31913277
- PMCID: PMC6949263
- DOI: 10.1038/s41467-019-13850-7
Prox1-positive cells monitor and sustain the murine intestinal epithelial cholinergic niche
Abstract
The enteric neurotransmitter acetylcholine governs important intestinal epithelial secretory and immune functions through its actions on epithelial muscarinic Gq-coupled receptors such as M3R. Its role in the regulation of intestinal stem cell function and differentiation, however, has not been clarified. Here, we find that nonselective muscarinic receptor antagonism in mice as well as epithelial-specific ablation of M3R induces a selective expansion of DCLK1-positive tuft cells, suggesting a model of feedback inhibition. Cholinergic blockade reduces Lgr5-positive intestinal stem cell tracing and cell number. In contrast, Prox1-positive endocrine cells appear as primary sensors of cholinergic blockade inducing the expansion of tuft cells, which adopt an enteroendocrine phenotype and contribute to increased mucosal levels of acetylcholine. This compensatory mechanism is lost with acute irradiation injury, resulting in a paucity of tuft cells and acetylcholine production. Thus, enteroendocrine tuft cells appear essential to maintain epithelial homeostasis following modifications of the cholinergic intestinal niche.
Conflict of interest statement
The authors declare no competing interests.
Figures
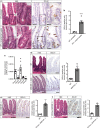
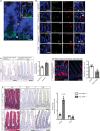
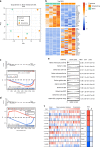
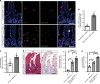
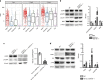
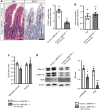
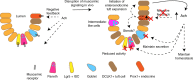
Similar articles
-
Tuft Cells and Their Role in Intestinal Diseases.Front Immunol. 2022 Feb 14;13:822867. doi: 10.3389/fimmu.2022.822867. eCollection 2022. Front Immunol. 2022. PMID: 35237268 Free PMC article. Review.
-
MicroRNA-195 regulates Tuft cell function in the intestinal epithelium by altering translation of DCLK1.Am J Physiol Cell Physiol. 2021 Jun 1;320(6):C1042-C1054. doi: 10.1152/ajpcell.00597.2020. Epub 2021 Mar 31. Am J Physiol Cell Physiol. 2021. PMID: 33788631 Free PMC article.
-
Dclk1-expressing tuft cells: critical modulators of the intestinal niche?Am J Physiol Gastrointest Liver Physiol. 2017 Oct 1;313(4):G285-G299. doi: 10.1152/ajpgi.00073.2017. Epub 2017 Jul 6. Am J Physiol Gastrointest Liver Physiol. 2017. PMID: 28684459 Free PMC article. Review.
-
Nerve Growth Factor Promotes Gastric Tumorigenesis through Aberrant Cholinergic Signaling.Cancer Cell. 2017 Jan 9;31(1):21-34. doi: 10.1016/j.ccell.2016.11.005. Epub 2016 Dec 15. Cancer Cell. 2017. PMID: 27989802 Free PMC article.
-
Nkx2.2 is expressed in a subset of enteroendocrine cells with expanded lineage potential.Am J Physiol Gastrointest Liver Physiol. 2015 Dec 15;309(12):G975-87. doi: 10.1152/ajpgi.00244.2015. Epub 2015 Oct 22. Am J Physiol Gastrointest Liver Physiol. 2015. PMID: 26492922 Free PMC article.
Cited by
-
Tuft cell-derived acetylcholine promotes epithelial chloride secretion and intestinal helminth clearance.Immunity. 2024 Jun 11;57(6):1243-1259.e8. doi: 10.1016/j.immuni.2024.03.023. Epub 2024 May 13. Immunity. 2024. PMID: 38744291
-
The Aging Enteric Nervous System.Int J Mol Sci. 2023 May 30;24(11):9471. doi: 10.3390/ijms24119471. Int J Mol Sci. 2023. PMID: 37298421 Free PMC article. Review.
-
Tuft cells are key mediators of interkingdom interactions at mucosal barrier surfaces.PLoS Pathog. 2022 Mar 10;18(3):e1010318. doi: 10.1371/journal.ppat.1010318. eCollection 2022 Mar. PLoS Pathog. 2022. PMID: 35271673 Free PMC article. Review.
-
Tuft Cells and Their Role in Intestinal Diseases.Front Immunol. 2022 Feb 14;13:822867. doi: 10.3389/fimmu.2022.822867. eCollection 2022. Front Immunol. 2022. PMID: 35237268 Free PMC article. Review.
-
WITHDRAWN: Gut Microbiota Alter Visceral Pain Sensation and Inflammation via Modulation of Synthesis of Resolvin D1 in Colonic Tuft Cells.Gastroenterology. 2022 Aug 4:S0016-5085(22)00829-0. doi: 10.1053/j.gastro.2022.07.053. Online ahead of print. Gastroenterology. 2022. PMID: 35934059 Free PMC article.
References
-
- Schemann M, Sann H, Schaaf C, Mader M. Identification of cholinergic neurons in enteric nervous system by antibodies against choline acetyltransferase. Am. J. Physiol. 1993;265:G1005–G1009. - PubMed
Publication types
MeSH terms
Substances
Grants and funding
LinkOut - more resources
Full Text Sources
Molecular Biology Databases