Genetically Engineering a Susceptible Mouse Model for MERS-CoV-Induced Acute Respiratory Distress Syndrome
- PMID: 31883094
- PMCID: PMC7123801
- DOI: 10.1007/978-1-0716-0211-9_12
Genetically Engineering a Susceptible Mouse Model for MERS-CoV-Induced Acute Respiratory Distress Syndrome
Abstract
Since 2012, monthly cases of Middle East respiratory syndrome coronavirus (MERS-CoV) continue to cause severe respiratory disease that is fatal in ~35% of diagnosed individuals. The ongoing threat to global public health and the need for novel therapeutic countermeasures have driven the development of animal models that can reproducibly replicate the pathology associated with MERS-CoV in human infections. The inability of MERS-CoV to replicate in the respiratory tracts of mice, hamsters, and ferrets stymied initial attempts to generate small animal models. Identification of human dipeptidyl peptidase IV (hDPP4) as the receptor for MERS-CoV infection opened the door for genetic engineering of mice. Precise molecular engineering of mouse DPP4 (mDPP4) with clustered regularly interspaced short palindromic repeats (CRISPR)/Cas9 technology maintained inherent expression profiles, and limited MERS-CoV susceptibility to tissues that naturally express mDPP4, notably the lower respiratory tract wherein MERS-CoV elicits severe pulmonary pathology. Here, we describe the generation of the 288-330+/+ MERS-CoV mouse model in which mice were made susceptible to MERS-CoV by modifying two amino acids on mDPP4 (A288 and T330), and the use of adaptive evolution to generate novel MERS-CoV isolates that cause fatal respiratory disease. The 288-330+/+ mice are currently being used to evaluate novel drug, antibody, and vaccine therapeutic countermeasures for MERS-CoV. The chapter starts with a historical perspective on the emergence of MERS-CoV and animal models evaluated for MERS-CoV pathogenesis, and then outlines the development of the 288-330+/+ mouse model, assays for assessing a MERS-CoV pulmonary infection in a mouse model, and describes some of the challenges associated with using genetically engineered mice.
Keywords: Cas9; Clustered regularly interspaced short palindromic repeats; Middle East respiratory syndrome coronavirus; Mouse; Pathogenesis.
Figures
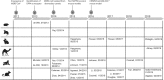
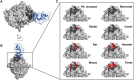
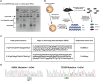
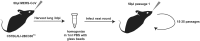
Similar articles
-
Acute Respiratory Infection in Human Dipeptidyl Peptidase 4-Transgenic Mice Infected with Middle East Respiratory Syndrome Coronavirus.J Virol. 2019 Mar 5;93(6):e01818-18. doi: 10.1128/JVI.01818-18. Print 2019 Mar 15. J Virol. 2019. PMID: 30626685 Free PMC article.
-
A mouse model for MERS coronavirus-induced acute respiratory distress syndrome.Nat Microbiol. 2016 Nov 28;2(2):16226. doi: 10.1038/nmicrobiol.2016.226. Nat Microbiol. 2016. PMID: 27892925 Free PMC article.
-
CD8+ T Cells and Macrophages Regulate Pathogenesis in a Mouse Model of Middle East Respiratory Syndrome.J Virol. 2016 Dec 16;91(1):e01825-16. doi: 10.1128/JVI.01825-16. Print 2017 Jan 1. J Virol. 2016. PMID: 27795435 Free PMC article.
-
Animal models of Middle East respiratory syndrome coronavirus infection.Antiviral Res. 2015 Oct;122:28-38. doi: 10.1016/j.antiviral.2015.07.005. Epub 2015 Jul 17. Antiviral Res. 2015. PMID: 26192750 Free PMC article. Review.
-
A Comparative Review of Animal Models of Middle East Respiratory Syndrome Coronavirus Infection.Vet Pathol. 2016 May;53(3):521-31. doi: 10.1177/0300985815620845. Epub 2016 Feb 11. Vet Pathol. 2016. PMID: 26869154 Review.
Cited by
-
Is 2020 the year when primatologists should cancel fieldwork?Am J Primatol. 2020 Aug;82(8):e23161. doi: 10.1002/ajp.23161. Epub 2020 Jun 24. Am J Primatol. 2020. PMID: 32583538 Free PMC article.
-
Coronavirus Disease 2019-COVID-19.Clin Microbiol Rev. 2020 Jun 24;33(4):e00028-20. doi: 10.1128/CMR.00028-20. Print 2020 Sep 16. Clin Microbiol Rev. 2020. PMID: 32580969 Free PMC article. Review.
-
Progresses in nucleic acid testing for COVID-19.Am J Transl Res. 2020 Oct 15;12(10):6763-6774. eCollection 2020. Am J Transl Res. 2020. PMID: 33194071 Free PMC article. Review.
-
COVID-19, an emerging coronavirus infection: advances and prospects in designing and developing vaccines, immunotherapeutics, and therapeutics.Hum Vaccin Immunother. 2020 Jun 2;16(6):1232-1238. doi: 10.1080/21645515.2020.1735227. Epub 2020 Mar 18. Hum Vaccin Immunother. 2020. PMID: 32186952 Free PMC article. Review.
-
Genetically modified mouse models to help fight COVID-19.Nat Protoc. 2020 Dec;15(12):3777-3787. doi: 10.1038/s41596-020-00403-2. Epub 2020 Oct 26. Nat Protoc. 2020. PMID: 33106680 Free PMC article. Review.
References
-
- Bernard-Stoecklin S, Nikolay B, Assiri A, Bin Saeed AA, Ben Embarek PK, El Bushra H, Ki M, Malik MR, Fontanet A, Cauchemez S, Van Kerkhove MD. Comparative analysis of eleven healthcare-associated outbreaks of Middle East respiratory syndrome coronavirus (Mers-Cov) from 2015 to 2017. Sci Rep. 2019;9:7385. doi: 10.1038/s41598-019-43586-9. - DOI - PMC - PubMed
MeSH terms
Substances
LinkOut - more resources
Full Text Sources
Molecular Biology Databases
Miscellaneous