Bioprinted Skin Recapitulates Normal Collagen Remodeling in Full-Thickness Wounds
- PMID: 31861970
- PMCID: PMC7249461
- DOI: 10.1089/ten.TEA.2019.0319
Bioprinted Skin Recapitulates Normal Collagen Remodeling in Full-Thickness Wounds
Abstract
Over 1 million burn injuries are treated annually in the United States, and current tissue engineered skin fails to meet the need for full-thickness replacement. Bioprinting technology has allowed fabrication of full-thickness skin and has demonstrated the ability to close full-thickness wounds. However, analysis of collagen remodeling in wounds treated with bioprinted skin has not been reported. The purpose of this study is to demonstrate the utility of bioprinted skin for epidermal barrier formation and normal collagen remodeling in full-thickness wounds. Human keratinocytes, melanocytes, fibroblasts, dermal microvascular endothelial cells, follicle dermal papilla cells, and adipocytes were suspended in fibrinogen bioink and bioprinted to form a tri-layer skin structure. Bioprinted skin was implanted onto 2.5 × 2.5 cm full-thickness excisional wounds on athymic mice, compared with wounds treated with hydrogel only or untreated wounds. Total wound closure, epithelialization, and contraction were quantified, and skin samples were harvested at 21 days for histology. Picrosirius red staining was used to quantify collagen fiber orientation, length, and width. Immunohistochemical (IHC) staining was performed to confirm epidermal barrier formation, dermal maturation, vascularity, and human cell integration. All bioprinted skin treated wounds closed by day 21, compared with open control wounds. Wound closure in bioprinted skin treated wounds was primarily due to epithelialization. In contrast, control hydrogel and untreated groups had sparse wound coverage and incomplete closure driven primarily by contraction. Picrosirius red staining confirmed a normal basket weave collagen organization in bioprinted skin-treated wounds compared with parallel collagen fibers in hydrogel only and untreated wounds. IHC staining at day 21 demonstrated the presence of human cells in the regenerated dermis, the formation of a stratified epidermis, dermal maturation, and blood vessel formation in bioprinted skin, none of which was present in control hydrogel treated wounds. Bioprinted skin accelerated full-thickness wound closure by promoting epidermal barrier formation, without increasing contraction. This healing process is associated with human cells from the bioprinted skin laying down a healthy, basket-weave collagen network. The remodeled skin is phenotypically similar to human skin and composed of a composite of graft and infiltrating host cells. Impact statement We have demonstrated the ability of bioprinted skin to enhance closure of full-thickness wounds through epithelialization and normal collagen remodeling. To our knowledge, this article is the first to quantify collagen remodeling by bioprinted skin in full-thickness wounds. Our methods and results can be used to guide further investigation of collagen remodeling by tissue engineered skin products to improve ongoing and future bioprinting skin studies. Ultimately, our skin bioprinting technology could translate into a new treatment for full-thickness wounds in human patients with the ability to recapitulate normal collagen remodeling in full-thickness wounds.
Keywords: animal models; bioprinting; extracellular matrix; skin; tissue engineering; wound healing.
Conflict of interest statement
No competing financial interests exist.
Figures
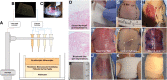
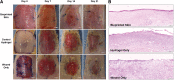
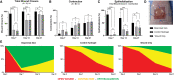
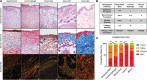
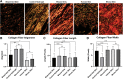
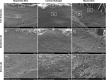
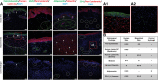
Similar articles
-
In Situ Bioprinting of Autologous Skin Cells Accelerates Wound Healing of Extensive Excisional Full-Thickness Wounds.Sci Rep. 2019 Feb 12;9(1):1856. doi: 10.1038/s41598-018-38366-w. Sci Rep. 2019. PMID: 30755653 Free PMC article.
-
Multicellular bioprinted skin facilitates human-like skin architecture in vivo.Sci Transl Med. 2023 Oct 4;15(716):eadf7547. doi: 10.1126/scitranslmed.adf7547. Epub 2023 Oct 4. Sci Transl Med. 2023. PMID: 37792956
-
Three Dimensional Bioprinting of a Vascularized and Perfusable Skin Graft Using Human Keratinocytes, Fibroblasts, Pericytes, and Endothelial Cells.Tissue Eng Part A. 2020 Mar;26(5-6):227-238. doi: 10.1089/ten.TEA.2019.0201. Epub 2019 Dec 3. Tissue Eng Part A. 2020. PMID: 31672103 Free PMC article.
-
Recent Advances in the Design of Three-Dimensional and Bioprinted Scaffolds for Full-Thickness Wound Healing.Tissue Eng Part B Rev. 2022 Feb;28(1):160-181. doi: 10.1089/ten.TEB.2020.0339. Epub 2021 Feb 22. Tissue Eng Part B Rev. 2022. PMID: 33446047 Review.
-
Cellular Interaction of Human Skin Cells towards Natural Bioink via 3D-Bioprinting Technologies for Chronic Wound: A Comprehensive Review.Int J Mol Sci. 2022 Jan 1;23(1):476. doi: 10.3390/ijms23010476. Int J Mol Sci. 2022. PMID: 35008902 Free PMC article. Review.
Cited by
-
3D skin bioprinting: future potential for skin regeneration.Postepy Dermatol Alergol. 2022 Oct;39(5):845-851. doi: 10.5114/ada.2021.109692. Epub 2021 Oct 4. Postepy Dermatol Alergol. 2022. PMID: 36457694 Free PMC article. Review.
-
Biopolymers for Tissue Engineering: Crosslinking, Printing Techniques, and Applications.Gels. 2023 Nov 10;9(11):890. doi: 10.3390/gels9110890. Gels. 2023. PMID: 37998980 Free PMC article. Review.
-
Bioprinting and plastic compression of large pigmented and vascularized human dermo-epidermal skin substitutes by means of a new robotic platform.J Tissue Eng. 2022 Apr 25;13:20417314221088513. doi: 10.1177/20417314221088513. eCollection 2022 Jan-Dec. J Tissue Eng. 2022. PMID: 35495096 Free PMC article.
-
Copper-Epigallocatechin Gallate Enhances Therapeutic Effects of 3D-Printed Dermal Scaffolds in Mitigating Diabetic Wound Scarring.ACS Appl Mater Interfaces. 2023 Aug 16;15(32):38230-38246. doi: 10.1021/acsami.3c04733. Epub 2023 Aug 3. ACS Appl Mater Interfaces. 2023. PMID: 37535406 Free PMC article.
-
Advances in the Research of Bioinks Based on Natural Collagen, Polysaccharide and Their Derivatives for Skin 3D Bioprinting.Polymers (Basel). 2020 May 29;12(6):1237. doi: 10.3390/polym12061237. Polymers (Basel). 2020. PMID: 32485901 Free PMC article. Review.
References
-
- American Burn Association. Burn incidence fact sheet. 2018. http://ameriburn.org/who-we-are/media/burn-incidence-fact-sheet (accessed November8, 2019)
-
- Peck M.D. Epidemiology of burns throughout the world. Part I: distribution and risk factors. Burns 37, 1087, 2011 - PubMed
-
- Bickers D.R., Lim H.W., Margolis D., et al. . The burden of skin diseases: 2004 a joint project of the American Academy of Dermatology Association and the Society for Investigative Dermatology. J Am Acad Dermatol 55, 490, 2006 - PubMed
Publication types
MeSH terms
Substances
Grants and funding
LinkOut - more resources
Full Text Sources
Other Literature Sources