Mechanisms of Homeostatic Synaptic Plasticity in vivo
- PMID: 31849610
- PMCID: PMC6901705
- DOI: 10.3389/fncel.2019.00520
Mechanisms of Homeostatic Synaptic Plasticity in vivo
Abstract
Synapses undergo rapid activity-dependent plasticity to store information, which when left uncompensated can lead to destabilization of neural function. It has been well documented that homeostatic changes, which operate at a slower time scale, are required to maintain stability of neural networks. While there are many mechanisms that can endow homeostatic control, sliding threshold and synaptic scaling are unique in that they operate by providing homeostatic control of synaptic strength. The former mechanism operates by adjusting the threshold for synaptic plasticity, while the latter mechanism directly alters the gain of synapses. Both modes of homeostatic synaptic plasticity have been studied across various preparations from reduced in vitro systems, such as neuronal cultures, to in vivo intact circuitry. While most of the cellular and molecular mechanisms of homeostatic synaptic plasticity have been worked out using reduced preparations, there are unique challenges present in intact circuitry in vivo, which deserve further consideration. For example, in an intact circuit, neurons receive distinct set of inputs across their dendritic tree which carry unique information. Homeostatic synaptic plasticity in vivo needs to operate without compromising processing of these distinct set of inputs to preserve information processing while maintaining network stability. In this mini review, we will summarize unique features of in vivo homeostatic synaptic plasticity, and discuss how sliding threshold and synaptic scaling may act across different activity regimes to provide homeostasis.
Keywords: BCM theory; cortical plasticity; hebbian plasticity; homeostasis; metaplasticity; sliding threshold; synaptic scaling.
Copyright © 2019 Lee and Kirkwood.
Figures
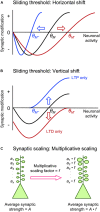
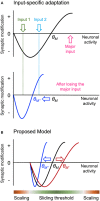
Similar articles
-
Disruption of NMDAR Function Prevents Normal Experience-Dependent Homeostatic Synaptic Plasticity in Mouse Primary Visual Cortex.J Neurosci. 2019 Sep 25;39(39):7664-7673. doi: 10.1523/JNEUROSCI.2117-18.2019. Epub 2019 Aug 14. J Neurosci. 2019. PMID: 31413075 Free PMC article.
-
Mechanisms of homeostatic plasticity in the excitatory synapse.J Neurochem. 2016 Dec;139(6):973-996. doi: 10.1111/jnc.13687. Epub 2016 Jul 1. J Neurochem. 2016. PMID: 27241695 Review.
-
Control of Homeostatic Synaptic Plasticity by AKAP-Anchored Kinase and Phosphatase Regulation of Ca2+-Permeable AMPA Receptors.J Neurosci. 2018 Mar 14;38(11):2863-2876. doi: 10.1523/JNEUROSCI.2362-17.2018. Epub 2018 Feb 13. J Neurosci. 2018. PMID: 29440558 Free PMC article.
-
Homeostatic Plasticity Achieved by Incorporation of Random Fluctuations and Soft-Bounded Hebbian Plasticity in Excitatory Synapses.Front Neural Circuits. 2016 Jun 1;10:42. doi: 10.3389/fncir.2016.00042. eCollection 2016. Front Neural Circuits. 2016. PMID: 27313513 Free PMC article.
-
Homeostatic role of heterosynaptic plasticity: models and experiments.Front Comput Neurosci. 2015 Jul 13;9:89. doi: 10.3389/fncom.2015.00089. eCollection 2015. Front Comput Neurosci. 2015. PMID: 26217218 Free PMC article. Review.
Cited by
-
Homeostatic Plasticity in Epilepsy.Front Cell Neurosci. 2020 Jun 26;14:197. doi: 10.3389/fncel.2020.00197. eCollection 2020. Front Cell Neurosci. 2020. PMID: 32676011 Free PMC article.
-
The Complex Formed by Group I Metabotropic Glutamate Receptor (mGluR) and Homer1a Plays a Central Role in Metaplasticity and Homeostatic Synaptic Scaling.J Neurosci. 2021 Jun 30;41(26):5567-5578. doi: 10.1523/JNEUROSCI.0026-21.2021. J Neurosci. 2021. PMID: 34193623 Free PMC article. Review.
-
Denervated mouse CA1 pyramidal neurons express homeostatic synaptic plasticity following entorhinal cortex lesion.Front Mol Neurosci. 2023 Apr 12;16:1148219. doi: 10.3389/fnmol.2023.1148219. eCollection 2023. Front Mol Neurosci. 2023. PMID: 37122623 Free PMC article.
-
Synchronous and opponent thermosensors use flexible cross-inhibition to orchestrate thermal homeostasis.Sci Adv. 2021 Aug 27;7(35):eabg6707. doi: 10.1126/sciadv.abg6707. Print 2021 Aug. Sci Adv. 2021. PMID: 34452914 Free PMC article.
-
Metaplasticity framework for cross-modal synaptic plasticity in adults.Front Synaptic Neurosci. 2023 Jan 6;14:1087042. doi: 10.3389/fnsyn.2022.1087042. eCollection 2022. Front Synaptic Neurosci. 2023. PMID: 36685084 Free PMC article. Review.
References
Publication types
Grants and funding
LinkOut - more resources
Full Text Sources
Research Materials