Ferritin is regulated by a neuro-intestinal axis in the nematode Caenorhabditis elegans
- PMID: 31677552
- PMCID: PMC6920132
- DOI: 10.1016/j.redox.2019.101359
Ferritin is regulated by a neuro-intestinal axis in the nematode Caenorhabditis elegans
Abstract
Iron is vital for the life of most organisms. However, when dysregulated, iron can catalyze the formation of oxygen (O2) radicals that can destroy any biological molecule and thus lead to oxidative injury and death. Therefore, iron metabolism must be tightly regulated at all times, as well as coordinated with the metabolism of O2. However, how is this achieved at the whole animal level is not well understood. Here, we explore this question using the nematode Caenorhabditis elegans. Exposure of worms to O2 starvation conditions (i.e. hypoxia) induces a major upregulation in levels of the conserved iron-cage protein ferritin 1 (ftn-1) in the intestine, while exposure to 21% O2 decreases ftn-1 level. This O2-dependent inhibition is mediated by O2-sensing neurons that communicate with the intestine through neurotransmitter and neuropeptide signalling, and requires the activity of hydroxylated HIF-1. By contrast, the induction of ftn-1 in hypoxia appears to be HIF-1-independent. This upregulation provides protection against Pseudomonas aeruginosa bacteria and oxidative injury. Taken together, our studies uncover a neuro-intestine axis that coordinates O2 and iron responses at the whole animal level.
Keywords: Caenorhabditis elegans; EGL-9; Ferritin; HIF-1; Hypoxia; Oxygen sensing neurons; Soluble guanylate cyclases; VHL-1; ftn-1.
Copyright © 2019 The Authors. Published by Elsevier B.V. All rights reserved.
Conflict of interest statement
The authors declare that they have no conflict of interest.
Figures
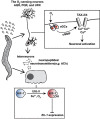
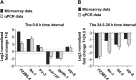
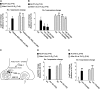
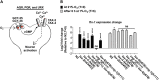
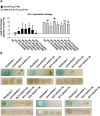
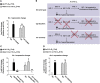
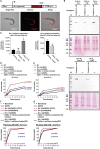
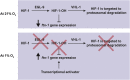
Similar articles
-
HIF-1 regulates iron homeostasis in Caenorhabditis elegans by activation and inhibition of genes involved in iron uptake and storage.PLoS Genet. 2011 Dec;7(12):e1002394. doi: 10.1371/journal.pgen.1002394. Epub 2011 Dec 15. PLoS Genet. 2011. PMID: 22194696 Free PMC article.
-
Synergism between soluble guanylate cyclase signaling and neuropeptides extends lifespan in the nematode Caenorhabditis elegans.Aging Cell. 2017 Apr;16(2):401-413. doi: 10.1111/acel.12569. Epub 2017 Jan 4. Aging Cell. 2017. PMID: 28054425 Free PMC article.
-
Insulin/IGF-1 and hypoxia signaling act in concert to regulate iron homeostasis in Caenorhabditis elegans.PLoS Genet. 2012;8(3):e1002498. doi: 10.1371/journal.pgen.1002498. Epub 2012 Mar 1. PLoS Genet. 2012. PMID: 22396654 Free PMC article.
-
Genetic analysis of hypoxia signaling and response in C elegans.Ann N Y Acad Sci. 2003 May;995:191-9. doi: 10.1111/j.1749-6632.2003.tb03222.x. Ann N Y Acad Sci. 2003. PMID: 12814951 Review.
-
The roles of neuropeptides in Caenorhabditis elegans including their importance in the regulation of feeding and metabolism.Protein Pept Lett. 2013 Jun;20(6):636-46. doi: 10.2174/0929866511320060004. Protein Pept Lett. 2013. PMID: 22630124 Review.
Cited by
-
Structural and functional relationship of mammalian and nematode ferritins.BioTechnologia (Pozn). 2021 Dec 22;102(4):457-471. doi: 10.5114/bta.2021.111110. eCollection 2021. BioTechnologia (Pozn). 2021. PMID: 36605605 Free PMC article. Review.
-
Distinct designer diamines promote mitophagy, and thereby enhance healthspan in C. elegans and protect human cells against oxidative damage.Autophagy. 2023 Feb;19(2):474-504. doi: 10.1080/15548627.2022.2078069. Epub 2022 Jun 1. Autophagy. 2023. PMID: 35579620 Free PMC article.
-
Role of ferroptosis in effects of anesthetics on multiple organ diseases: A literature review.Heliyon. 2023 Sep 23;9(10):e20405. doi: 10.1016/j.heliyon.2023.e20405. eCollection 2023 Oct. Heliyon. 2023. PMID: 37780755 Free PMC article. Review.
-
Transcriptome analyses describe the consequences of persistent HIF-1 over-activation in Caenorhabditis elegans.PLoS One. 2024 Mar 22;19(3):e0295093. doi: 10.1371/journal.pone.0295093. eCollection 2024. PLoS One. 2024. PMID: 38517909 Free PMC article.
-
Allele-specific mitochondrial stress induced by Multiple Mitochondrial Dysfunctions Syndrome 1 pathogenic mutations modeled in Caenorhabditis elegans.PLoS Genet. 2021 Aug 27;17(8):e1009771. doi: 10.1371/journal.pgen.1009771. eCollection 2021 Aug. PLoS Genet. 2021. PMID: 34449775 Free PMC article.
References
-
- Halliwell Barry, John G. In: Free Radicals in Biology and Medicine. Gutteridge John., editor. Clarendon Press; Oxford: 2007.
-
- Winterbourn C.C. Toxicity of iron and hydrogen peroxide: the Fenton reaction. Toxicol. Lett. 1995;82–83:969–974. - PubMed
-
- Chepelev N.L., Willmore W.G. Regulation of iron pathways in response to hypoxia. Free Radic. Biol. Med. 2011;50(6):645–666. - PubMed
-
- Dixon S.J., Stockwell B.R. The role of iron and reactive oxygen species in cell death. Nat. Chem. Biol. 2014;10(1):9–17. - PubMed
Publication types
MeSH terms
Substances
Grants and funding
LinkOut - more resources
Full Text Sources
Molecular Biology Databases