Three Dimensional Bioprinting of a Vascularized and Perfusable Skin Graft Using Human Keratinocytes, Fibroblasts, Pericytes, and Endothelial Cells
- PMID: 31672103
- PMCID: PMC7476394
- DOI: 10.1089/ten.TEA.2019.0201
Three Dimensional Bioprinting of a Vascularized and Perfusable Skin Graft Using Human Keratinocytes, Fibroblasts, Pericytes, and Endothelial Cells
Abstract
Multilayered skin substitutes comprising allogeneic cells have been tested for the treatment of nonhealing cutaneous ulcers. However, such nonnative skin grafts fail to permanently engraft because they lack dermal vascular networks important for integration with the host tissue. In this study, we describe the fabrication of an implantable multilayered vascularized bioengineered skin graft using 3D bioprinting. The graft is formed using one bioink containing human foreskin dermal fibroblasts (FBs), human endothelial cells (ECs) derived from cord blood human endothelial colony-forming cells (HECFCs), and human placental pericytes (PCs) suspended in rat tail type I collagen to form a dermis followed by printing with a second bioink containing human foreskin keratinocytes (KCs) to form an epidermis. In vitro, KCs replicate and mature to form a multilayered barrier, while the ECs and PCs self-assemble into interconnected microvascular networks. The PCs in the dermal bioink associate with EC-lined vascular structures and appear to improve KC maturation. When these 3D printed grafts are implanted on the dorsum of immunodeficient mice, the human EC-lined structures inosculate with mouse microvessels arising from the wound bed and become perfused within 4 weeks after implantation. The presence of PCs in the printed dermis enhances the invasion of the graft by host microvessels and the formation of an epidermal rete. Impact Statement Three Dimensional printing can be used to generate multilayered vascularized human skin grafts that can potentially overcome the limitations of graft survival observed in current avascular skin substitutes. Inclusion of human pericytes in the dermal bioink appears to improve both dermal and epidermal maturation.
Keywords: bioprinting; microvasculature; regenerative medicine; skin; tissue engineering.
Conflict of interest statement
No competing financial interests exist.
Figures
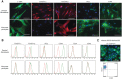
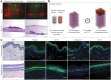
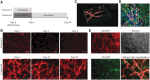
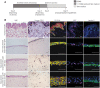
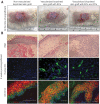
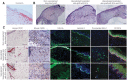
Similar articles
-
3D bioprinting of an implantable xeno-free vascularized human skin graft.Bioeng Transl Med. 2022 Apr 21;8(1):e10324. doi: 10.1002/btm2.10324. eCollection 2023 Jan. Bioeng Transl Med. 2022. PMID: 36684084 Free PMC article.
-
Bioprinted Skin Recapitulates Normal Collagen Remodeling in Full-Thickness Wounds.Tissue Eng Part A. 2020 May;26(9-10):512-526. doi: 10.1089/ten.TEA.2019.0319. Epub 2020 Jan 28. Tissue Eng Part A. 2020. PMID: 31861970 Free PMC article.
-
3D bioprinting of functional human skin: production and in vivo analysis.Biofabrication. 2016 Dec 5;9(1):015006. doi: 10.1088/1758-5090/9/1/015006. Biofabrication. 2016. PMID: 27917823
-
Dermal Contributions to Human Interfollicular Epidermal Architecture and Self-Renewal.Int J Mol Sci. 2015 Nov 25;16(12):28098-107. doi: 10.3390/ijms161226078. Int J Mol Sci. 2015. PMID: 26602926 Free PMC article. Review.
-
Cellular Interaction of Human Skin Cells towards Natural Bioink via 3D-Bioprinting Technologies for Chronic Wound: A Comprehensive Review.Int J Mol Sci. 2022 Jan 1;23(1):476. doi: 10.3390/ijms23010476. Int J Mol Sci. 2022. PMID: 35008902 Free PMC article. Review.
Cited by
-
Organotypic cultures as aging associated disease models.Aging (Albany NY). 2022 Nov 22;14(22):9338-9383. doi: 10.18632/aging.204361. Epub 2022 Nov 22. Aging (Albany NY). 2022. PMID: 36435511 Free PMC article. Review.
-
A Concise Review on Tissue Engineered Artificial Skin Grafts for Chronic Wound Treatment: Can We Reconstruct Functional Skin Tissue In Vitro?Cells. 2020 Jul 6;9(7):1622. doi: 10.3390/cells9071622. Cells. 2020. PMID: 32640572 Free PMC article. Review.
-
Bioprinting and plastic compression of large pigmented and vascularized human dermo-epidermal skin substitutes by means of a new robotic platform.J Tissue Eng. 2022 Apr 25;13:20417314221088513. doi: 10.1177/20417314221088513. eCollection 2022 Jan-Dec. J Tissue Eng. 2022. PMID: 35495096 Free PMC article.
-
Bioinks Enriched with ECM Components Obtained by Supercritical Extraction.Biomolecules. 2022 Mar 2;12(3):394. doi: 10.3390/biom12030394. Biomolecules. 2022. PMID: 35327586 Free PMC article.
-
Impact of Cell Seeding Density and Cell Confluence on Human Tissue Engineered Skeletal Muscle.Tissue Eng Part A. 2022 May;28(9-10):420-432. doi: 10.1089/ten.TEA.2021.0132. Epub 2022 Feb 23. Tissue Eng Part A. 2022. PMID: 34652973 Free PMC article.
References
-
- Singer A.J., and Clark R.A.F.. Cutaneous wound healing. N Engl J Med 341, 738, 1999 - PubMed
-
- Falanga V. Wound healing and its impairment in the diabetic foot. Lancet 366, 1736, 2005 - PubMed
-
- Falanga V. The chronic wound: impaired healing and solutions in the context of wound bed preparation. Blood Cells Mol Dis 32, 88, 2004 - PubMed
Publication types
MeSH terms
Substances
Grants and funding
LinkOut - more resources
Full Text Sources
Other Literature Sources