Effects of interleukin-2 in immunostimulation and immunosuppression
- PMID: 31611250
- PMCID: PMC7037245
- DOI: 10.1084/jem.20191247
Effects of interleukin-2 in immunostimulation and immunosuppression
Abstract
Historically, interleukin-2 (IL-2) was first described as an immunostimulatory factor that supports the expansion of activated effector T cells. A layer of sophistication arose when regulatory CD4+ T lymphocytes (Tregs) were shown to require IL-2 for their development, homeostasis, and immunosuppressive functions. Fundamental distinctions in the nature and spatiotemporal expression patterns of IL-2 receptor subunits on naive/memory/effector T cells versus Tregs are now being exploited to manipulate the immunomodulatory effects of IL-2 for therapeutic purposes. Although high-dose IL-2 administration has yielded discrete clinical responses, low-dose IL-2 as well as innovative strategies based on IL-2 derivatives, including "muteins," immunocomplexes, and immunocytokines, are being explored to therapeutically enhance or inhibit the immune response.
© 2019 Pol et al.
Figures
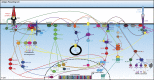
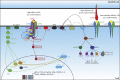
Similar articles
-
Tolerance, not immunity, crucially depends on IL-2.Nat Rev Immunol. 2004 Sep;4(9):665-74. doi: 10.1038/nri1435. Nat Rev Immunol. 2004. PMID: 15343366 Review.
-
Stimulation of α7 nicotinic acetylcholine receptor by nicotine increases suppressive capacity of naturally occurring CD4+CD25+ regulatory T cells in mice in vitro.J Pharmacol Exp Ther. 2010 Dec;335(3):553-61. doi: 10.1124/jpet.110.169961. Epub 2010 Sep 15. J Pharmacol Exp Ther. 2010. PMID: 20843956
-
IL-15 and cognate antigen successfully expand de novo-induced human antigen-specific regulatory CD4+ T cells that require antigen-specific activation for suppression.J Immunol. 2003 Dec 15;171(12):6431-41. doi: 10.4049/jimmunol.171.12.6431. J Immunol. 2003. PMID: 14662842
-
Tolerogenic dendritic cells inhibit antiphospholipid syndrome derived effector/memory CD4⁺ T cell response to β2GPI.Ann Rheum Dis. 2012 Jan;71(1):120-8. doi: 10.1136/annrheumdis-2011-200063. Epub 2011 Sep 12. Ann Rheum Dis. 2012. PMID: 21914629
-
Opposing functions of IL-2 and IL-7 in the regulation of immune responses.Cytokine. 2011 Oct;56(1):116-21. doi: 10.1016/j.cyto.2011.07.005. Epub 2011 Jul 31. Cytokine. 2011. PMID: 21807532 Free PMC article. Review.
Cited by
-
Diminished Pneumococcal-Specific CD4+ T-Cell Response is Associated With Increased Regulatory T Cells at Older Age.Front Aging. 2021 Nov 3;2:746295. doi: 10.3389/fragi.2021.746295. eCollection 2021. Front Aging. 2021. PMID: 35822055 Free PMC article.
-
Oncolytic virus promotes tumor-reactive infiltrating lymphocytes for adoptive cell therapy.Cancer Gene Ther. 2021 Feb;28(1-2):98-111. doi: 10.1038/s41417-020-0189-4. Epub 2020 Jul 7. Cancer Gene Ther. 2021. PMID: 32632271 Free PMC article.
-
Understanding the feasibility of chemotherapeutic and immunotherapeutic targets against non-small cell lung cancers: an update of resistant responses and recent combinatorial therapies.Explor Target Antitumor Ther. 2023;4(5):850-895. doi: 10.37349/etat.2023.00171. Epub 2023 Oct 10. Explor Target Antitumor Ther. 2023. PMID: 37970206 Free PMC article. Review.
-
Regulatory T cells as a therapeutic approach for inflammatory bowel disease.Eur J Immunol. 2023 Feb;53(2):e2250007. doi: 10.1002/eji.202250007. Epub 2023 Jan 6. Eur J Immunol. 2023. PMID: 36562391 Free PMC article. Review.
-
A Protease Activatable Interleukin-2 Fusion Protein Engenders Antitumor Immune Responses by Interferon Gamma-Dependent and Interferon Gamma-Independent Mechanisms.J Interferon Cytokine Res. 2022 Jul;42(7):316-328. doi: 10.1089/jir.2022.0043. J Interferon Cytokine Res. 2022. PMID: 35834651 Free PMC article.
References
-
- Allison J.P., McIntyre B.W., and Bloch D.. 1982. Tumor-specific antigen of murine T-lymphoma defined with monoclonal antibody. J. Immunol. 129:2293–2300. - PubMed
-
- Alva A., Daniels G.A., Wong M.K., Kaufman H.L., Morse M.A., McDermott D.F., Clark J.I., Agarwala S.S., Miletello G., Logan T.F., et al. . 2016. Contemporary experience with high-dose interleukin-2 therapy and impact on survival in patients with metastatic melanoma and metastatic renal cell carcinoma. Cancer Immunol. Immunother. 65:1533–1544. 10.1007/s00262-016-1910-x - DOI - PMC - PubMed
-
- Andreu-Sánchez J.L., Moreno de Alborán I.M., Marcos M.A., Sánchez-Movilla A., Martínez-A C., and Kroemer G.. 1991. Interleukin 2 abrogates the nonresponsive state of T cells expressing a forbidden T cell receptor repertoire and induces autoimmune disease in neonatally thymectomized mice. J. Exp. Med. 173:1323–1329. 10.1084/jem.173.6.1323 - DOI - PMC - PubMed
-
- Arenas-Ramirez N., Zou C., Popp S., Zingg D., Brannetti B., Wirth E., Calzascia T., Kovarik J., Sommer L., Zenke G., et al. . 2016. Improved cancer immunotherapy by a CD25-mimobody conferring selectivity to human interleukin-2. Sci. Transl. Med. 8:367ra166 10.1126/scitranslmed.aag3187 - DOI - PubMed
Publication types
MeSH terms
Substances
LinkOut - more resources
Full Text Sources
Other Literature Sources
Research Materials