CAF hierarchy driven by pancreatic cancer cell p53-status creates a pro-metastatic and chemoresistant environment via perlecan
- PMID: 31406163
- PMCID: PMC6691013
- DOI: 10.1038/s41467-019-10968-6
CAF hierarchy driven by pancreatic cancer cell p53-status creates a pro-metastatic and chemoresistant environment via perlecan
Abstract
Heterogeneous subtypes of cancer-associated fibroblasts (CAFs) coexist within pancreatic cancer tissues and can both promote and restrain disease progression. Here, we interrogate how cancer cells harboring distinct alterations in p53 manipulate CAFs. We reveal the existence of a p53-driven hierarchy, where cancer cells with a gain-of-function (GOF) mutant p53 educate a dominant population of CAFs that establish a pro-metastatic environment for GOF and null p53 cancer cells alike. We also demonstrate that CAFs educated by null p53 cancer cells may be reprogrammed by either GOF mutant p53 cells or their CAFs. We identify perlecan as a key component of this pro-metastatic environment. Using intravital imaging, we observe that these dominant CAFs delay cancer cell response to chemotherapy. Lastly, we reveal that depleting perlecan in the stroma combined with chemotherapy prolongs mouse survival, supporting it as a potential target for anti-stromal therapies in pancreatic cancer.
Conflict of interest statement
The authors declare no competing interests.
Figures
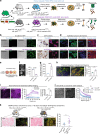
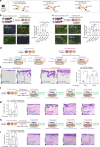
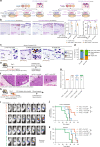
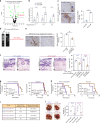
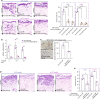
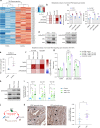
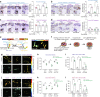
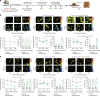
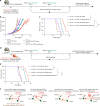
Comment in
-
Targeting genetically-tuned CAFs in pancreatic cancer via perlecan manipulation.Expert Opin Ther Targets. 2020 Mar;24(3):171-174. doi: 10.1080/14728222.2020.1727887. Epub 2020 Feb 13. Expert Opin Ther Targets. 2020. PMID: 32031028
Similar articles
-
Targeting genetically-tuned CAFs in pancreatic cancer via perlecan manipulation.Expert Opin Ther Targets. 2020 Mar;24(3):171-174. doi: 10.1080/14728222.2020.1727887. Epub 2020 Feb 13. Expert Opin Ther Targets. 2020. PMID: 32031028
-
CD146 attenuation in cancer-associated fibroblasts promotes pancreatic cancer progression.Mol Carcinog. 2016 Nov;55(11):1560-1572. doi: 10.1002/mc.22409. Epub 2015 Sep 16. Mol Carcinog. 2016. PMID: 26373617
-
Chemotherapy-Induced Inflammatory Gene Signature and Protumorigenic Phenotype in Pancreatic CAFs via Stress-Associated MAPK.Mol Cancer Res. 2016 May;14(5):437-47. doi: 10.1158/1541-7786.MCR-15-0348. Epub 2016 Mar 15. Mol Cancer Res. 2016. PMID: 26979711 Free PMC article.
-
Recent advances in understanding cancer-associated fibroblasts in pancreatic cancer.Am J Physiol Cell Physiol. 2020 Aug 1;319(2):C233-C243. doi: 10.1152/ajpcell.00079.2020. Epub 2020 May 20. Am J Physiol Cell Physiol. 2020. PMID: 32432930 Free PMC article. Review.
-
Regulation of heterogeneous cancer-associated fibroblasts: the molecular pathology of activated signaling pathways.J Exp Clin Cancer Res. 2020 Jun 16;39(1):112. doi: 10.1186/s13046-020-01611-0. J Exp Clin Cancer Res. 2020. PMID: 32546182 Free PMC article. Review.
Cited by
-
Cancer-associated fibroblasts: overview, progress, challenges, and directions.Cancer Gene Ther. 2021 Sep;28(9):984-999. doi: 10.1038/s41417-021-00318-4. Epub 2021 Mar 12. Cancer Gene Ther. 2021. PMID: 33712707 Review.
-
Cancer-Associated Fibroblast-Induced Resistance to Chemotherapy and Radiotherapy in Gastrointestinal Cancers.Cancers (Basel). 2021 Mar 9;13(5):1172. doi: 10.3390/cancers13051172. Cancers (Basel). 2021. PMID: 33803229 Free PMC article. Review.
-
Mutant p53 in cell-cell interactions.Genes Dev. 2021 Apr 1;35(7-8):433-448. doi: 10.1101/gad.347542.120. Genes Dev. 2021. PMID: 33861719 Free PMC article. Review.
-
Temporally resolved proteomics identifies nidogen-2 as a cotarget in pancreatic cancer that modulates fibrosis and therapy response.Sci Adv. 2024 Jul 5;10(27):eadl1197. doi: 10.1126/sciadv.adl1197. Epub 2024 Jul 3. Sci Adv. 2024. PMID: 38959305 Free PMC article.
-
Distance-depending transcriptome changes of pancreatic stellate cells in paracrine pancreatic ductal adenocarcinoma co-culture models.Sci Rep. 2024 Aug 4;14(1):18030. doi: 10.1038/s41598-024-68148-6. Sci Rep. 2024. PMID: 39098880 Free PMC article.
References
Publication types
MeSH terms
Substances
Grants and funding
LinkOut - more resources
Full Text Sources
Medical
Molecular Biology Databases
Research Materials
Miscellaneous