Whole-animal connectomes of both Caenorhabditis elegans sexes
- PMID: 31270481
- PMCID: PMC6889226
- DOI: 10.1038/s41586-019-1352-7
Whole-animal connectomes of both Caenorhabditis elegans sexes
Abstract
Knowledge of connectivity in the nervous system is essential to understanding its function. Here we describe connectomes for both adult sexes of the nematode Caenorhabditis elegans, an important model organism for neuroscience research. We present quantitative connectivity matrices that encompass all connections from sensory input to end-organ output across the entire animal, information that is necessary to model behaviour. Serial electron microscopy reconstructions that are based on the analysis of both new and previously published electron micrographs update previous results and include data on the male head. The nervous system differs between sexes at multiple levels. Several sex-shared neurons that function in circuits for sexual behaviour are sexually dimorphic in structure and connectivity. Inputs from sex-specific circuitry to central circuitry reveal points at which sexual and non-sexual pathways converge. In sex-shared central pathways, a substantial number of connections differ in strength between the sexes. Quantitative connectomes that include all connections serve as the basis for understanding how complex, adaptive behavior is generated.
Figures
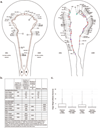
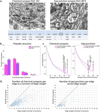
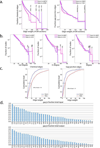
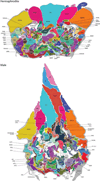
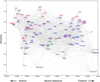
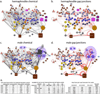
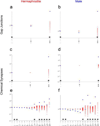
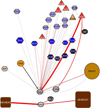
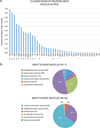
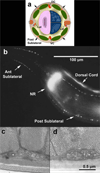
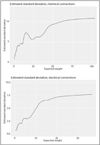
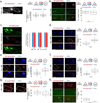
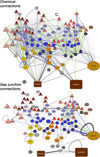
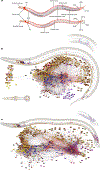
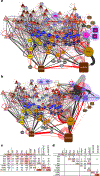
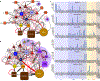
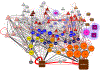
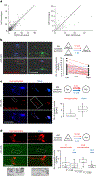
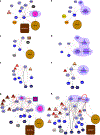
Comment in
-
Neural networks mapped in both sexes of the worm.Nature. 2019 Jul;571(7763):40-42. doi: 10.1038/d41586-019-02006-8. Nature. 2019. PMID: 31270489 No abstract available.
Similar articles
-
Neural Circuits of Sexual Behavior in Caenorhabditis elegans.Annu Rev Neurosci. 2018 Jul 8;41:349-369. doi: 10.1146/annurev-neuro-070815-014056. Epub 2018 Apr 25. Annu Rev Neurosci. 2018. PMID: 29709211 Review.
-
What about the males? the C. elegans sexually dimorphic nervous system and a CRISPR-based tool to study males in a hermaphroditic species.J Neurogenet. 2020 Sep-Dec;34(3-4):323-334. doi: 10.1080/01677063.2020.1789978. Epub 2020 Jul 10. J Neurogenet. 2020. PMID: 32648491 Free PMC article.
-
Sexual modulation of sex-shared neurons and circuits in Caenorhabditis elegans.J Neurosci Res. 2017 Jan 2;95(1-2):527-538. doi: 10.1002/jnr.23912. J Neurosci Res. 2017. PMID: 27870393 Free PMC article. Review.
-
A Pipeline for Volume Electron Microscopy of the Caenorhabditis elegans Nervous System.Front Neural Circuits. 2018 Nov 21;12:94. doi: 10.3389/fncir.2018.00094. eCollection 2018. Front Neural Circuits. 2018. PMID: 30524248 Free PMC article.
-
Computer assisted assembly of connectomes from electron micrographs: application to Caenorhabditis elegans.PLoS One. 2013;8(1):e54050. doi: 10.1371/journal.pone.0054050. Epub 2013 Jan 16. PLoS One. 2013. PMID: 23342070 Free PMC article.
Cited by
-
Sexually dimorphic architecture and function of a mechanosensory circuit in C. elegans.Nat Commun. 2022 Nov 11;13(1):6825. doi: 10.1038/s41467-022-34661-3. Nat Commun. 2022. PMID: 36369281 Free PMC article.
-
Amyotrophic Lateral Sclerosis Mechanism: Insights from the Caenorhabditis elegans Models.Cells. 2024 Jan 3;13(1):99. doi: 10.3390/cells13010099. Cells. 2024. PMID: 38201303 Free PMC article. Review.
-
The Wiring Logic of an Identified Serotonergic Neuron That Spans Sensory Networks.J Neurosci. 2020 Aug 12;40(33):6309-6327. doi: 10.1523/JNEUROSCI.0552-20.2020. Epub 2020 Jul 8. J Neurosci. 2020. PMID: 32641403 Free PMC article.
-
Probing fine-scale connections in the brain.Nature. 2020 Oct;586(7830):631-633. doi: 10.1038/d41586-020-02947-5. Nature. 2020. PMID: 33077941 No abstract available.
-
The relationship between intraflagellar transport and upstream protein trafficking pathways and macrocyclic lactone resistance in Caenorhabditis elegans.G3 (Bethesda). 2024 Mar 6;14(3):jkae009. doi: 10.1093/g3journal/jkae009. G3 (Bethesda). 2024. PMID: 38227795 Free PMC article.
References
-
- White JG, Southgate E, Thomson JN & Brenner S The structure of the nervous system of the nematode Caenorhabditis elegans. Phil. Trans. R. Soc. Lond. B 314, 1–340 (1986). - PubMed
-
- Albertson DG & Thomson JN The pharynx of Caenorhabditis elegans. Phil. Trans. R. Soc. Lond. B 275, 299–325 (1976). - PubMed
-
- Jarrell TA et al. The connectome of a decision-making neural network. Science 337, 437–444 (2012). - PubMed
-
- Bumbarger DJ, Riebesell M, Rödelsperger C & Sommer RJ System-wide rewiring underlies behavioral differences in predatory and bacterial-feeding nematodes. Cell 152, 109–119 (2013). - PubMed
Publication types
MeSH terms
Grants and funding
LinkOut - more resources
Full Text Sources