Pannexin 1 Regulates Network Ensembles and Dendritic Spine Development in Cortical Neurons
- PMID: 31118206
- PMCID: PMC6557035
- DOI: 10.1523/ENEURO.0503-18.2019
Pannexin 1 Regulates Network Ensembles and Dendritic Spine Development in Cortical Neurons
Abstract
Dendritic spines are the postsynaptic targets of excitatory synaptic inputs that undergo extensive proliferation and maturation during the first postnatal month in mice. However, our understanding of the molecular mechanisms that regulate spines during this critical period is limited. Previous work has shown that pannexin 1 (Panx1) regulates neurite growth and synaptic plasticity. We therefore investigated the impact of global Panx1 KO on spontaneous cortical neuron activity using Ca2+ imaging and in silico network analysis. Panx1 KO increased both the number and size of spontaneous co-active cortical neuron network ensembles. To understand the basis for these findings, we investigated Panx1 expression in postnatal synaptosome preparations from early postnatal mouse cortex. Between 2 and 4 postnatal weeks, we observed a precipitous drop in cortical synaptosome protein levels of Panx1, suggesting it regulates synapse proliferation and/or maturation. At the same time points, we observed significant enrichment of the excitatory postsynaptic density proteins PSD-95, GluA1, and GluN2a in cortical synaptosomes from global Panx1 knock-out mice. Ex vivo analysis of pyramidal neuron structure in somatosensory cortex revealed a consistent increase in dendritic spine densities in both male and female Panx1 KO mice. Similar findings were observed in an excitatory neuron-specific Panx1 KO line (Emx1-Cre driven; Panx1 cKOE) and in primary Panx1 KO cortical neurons cultured in vitro. Altogether, our study suggests that Panx1 negatively regulates cortical dendritic spine development.
Keywords: cortical neuron; critical period; dendritic spines; network ensembles; pannexin; somatosensory.
Copyright © 2019 Sanchez-Arias et al.
Figures
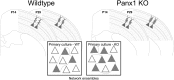
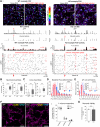
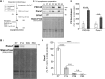
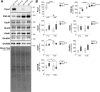
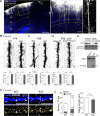
Similar articles
-
Close Homolog of L1 Regulates Dendritic Spine Density in the Mouse Cerebral Cortex Through Semaphorin 3B.J Neurosci. 2019 Aug 7;39(32):6233-6250. doi: 10.1523/JNEUROSCI.2984-18.2019. Epub 2019 Jun 10. J Neurosci. 2019. PMID: 31182634 Free PMC article.
-
Pannexin 1 Regulates Dendritic Protrusion Dynamics in Immature Cortical Neurons.eNeuro. 2020 Aug 26;7(4):ENEURO.0079-20.2020. doi: 10.1523/ENEURO.0079-20.2020. Print 2020 Jul/Aug. eNeuro. 2020. PMID: 32737184 Free PMC article.
-
mGluR5 Exerts Cell-Autonomous Influences on the Functional and Anatomical Development of Layer IV Cortical Neurons in the Mouse Primary Somatosensory Cortex.J Neurosci. 2016 Aug 24;36(34):8802-14. doi: 10.1523/JNEUROSCI.1224-16.2016. J Neurosci. 2016. PMID: 27559164 Free PMC article.
-
Cortical dendritic spine development and plasticity: insights from in vivo imaging.Curr Opin Neurobiol. 2018 Dec;53:76-82. doi: 10.1016/j.conb.2018.06.002. Epub 2018 Jun 21. Curr Opin Neurobiol. 2018. PMID: 29936406 Review.
-
Spine Dynamics: Are They All the Same?Neuron. 2017 Sep 27;96(1):43-55. doi: 10.1016/j.neuron.2017.08.008. Neuron. 2017. PMID: 28957675 Free PMC article. Review.
Cited by
-
ER-resident STIM1/2 couples Ca2+ entry by NMDA receptors to pannexin-1 activation.Proc Natl Acad Sci U S A. 2022 Sep 6;119(36):e2112870119. doi: 10.1073/pnas.2112870119. Epub 2022 Aug 29. Proc Natl Acad Sci U S A. 2022. PMID: 36037373 Free PMC article.
-
Overlap in synaptic neurological condition susceptibility pathways and the neural pannexin 1 interactome revealed by bioinformatics analyses.Channels (Austin). 2023 Dec;17(1):2253102. doi: 10.1080/19336950.2023.2253102. Epub 2023 Oct 8. Channels (Austin). 2023. PMID: 37807670 Free PMC article.
-
Pannexin 1 channels and ATP release in epilepsy: two sides of the same coin : The contribution of pannexin-1, connexins, and CALHM ATP-release channels to purinergic signaling.Purinergic Signal. 2021 Dec;17(4):533-548. doi: 10.1007/s11302-021-09818-2. Epub 2021 Sep 8. Purinergic Signal. 2021. PMID: 34495463 Free PMC article. Review.
-
Pannexin 1 Influences Lineage Specification of Human iPSCs.Front Cell Dev Biol. 2021 Apr 16;9:659397. doi: 10.3389/fcell.2021.659397. eCollection 2021. Front Cell Dev Biol. 2021. PMID: 33937260 Free PMC article.
-
Ankyrin B and Ankyrin B variants differentially modulate intracellular and surface Cav2.1 levels.Mol Brain. 2019 Sep 2;12(1):75. doi: 10.1186/s13041-019-0494-8. Mol Brain. 2019. PMID: 31477143 Free PMC article.
References
-
- Awad PN, Amegandjin CA, Szczurkowska J, Carriço JN, Fernandes do Nascimento AS, Baho E, Chattopadhyaya B, Cancedda L, Carmant L, Di Cristo G (2018) KCC2 regulates dendritic spine formation in a brain-region specific and BDNF dependent manner. Cereb Cortex 28:4049–4062. 10.1093/cercor/bhy198 - DOI - PMC - PubMed
-
- Bardy C, Hurk M van den, Eames T, Marchand C, Hernandez RV, Kellogg M, Gorris M, Galet B, Palomares V, Brown J, Bang AG, Mertens J, Böhnke L, Boyer L, Simon S, Gage FH (2015) Neuronal medium that supports basic synaptic functions and activity of human neurons in vitro. Proc Natl Acad Sci USA 112:E2725–E2734. 10.1073/pnas.1504393112 - DOI - PMC - PubMed
Publication types
MeSH terms
Substances
LinkOut - more resources
Full Text Sources
Molecular Biology Databases
Research Materials
Miscellaneous