Clearance of damaged mitochondria via mitophagy is important to the protective effect of ischemic preconditioning in kidneys
- PMID: 31066324
- PMCID: PMC6844514
- DOI: 10.1080/15548627.2019.1615822
Clearance of damaged mitochondria via mitophagy is important to the protective effect of ischemic preconditioning in kidneys
Abstract
Ischemic preconditioning (IPC) affords tissue protection in organs including kidneys; however, the underlying mechanism remains unclear. Here we demonstrate an important role of macroautophagy/autophagy (especially mitophagy) in the protective effect of IPC in kidneys. IPC induced autophagy in renal tubular cells in mice and suppressed subsequent renal ischemia-reperfusion injury (IRI). The protective effect of IPC was abolished by pharmacological inhibitors of autophagy and by the ablation of Atg7 from kidney proximal tubules. Pretreatment with BECN1/Beclin1 peptide induced autophagy and protected against IRI. These results suggest the dependence of IPC protection on renal autophagy. During IPC, the mitophagy regulator PINK1 (PTEN induced putative kinase 1) was activated. Both IPC and BECN1 peptide enhanced mitolysosome formation during renal IRI in mitophagy reporter mice, suggesting that IPC may protect kidneys by activating mitophagy. We further established an in vitro model of IPC by inducing 'chemical ischemia' in kidney proximal tubular cells with carbonyl cyanide 3-chlorophenylhydrazone (CCCP). Brief treatment with CCCP protected against subsequent injury in these cells and the protective effect was abrogated by autophagy inhibition. In vitro IPC increased mitophagosome formation, enhanced the delivery of mitophagosomes to lysosomes, and promoted the clearance of damaged mitochondria during subsequent CCCP treatment. IPC also suppressed mitochondrial depolarization, improved ATP production, and inhibited the generation of reactive oxygen species. Knockdown of Pink1 suppressed mitophagy and reduced the cytoprotective effect of IPC. Together, these results suggest that autophagy, especially mitophagy, plays an important role in the protective effect of IPC.Abbreviations: ACTB: actin, beta; ATG: autophagy related; BNIP3: BCL2 interacting protein 3; BNIP3L/NIX: BCL2 interacting protein 3 like; BUN: blood urea nitrogen; CASP3: caspase 3; CCCP: carbonyl cyanide 3-chlorophenylhydrazone; COX4I1: cytochrome c oxidase subunit 4I1; COX8: cytochrome c oxidase subunit 8; DAPI: 4',6-diamidino-2-phenylindole; DNM1L: dynamin 1 like; EGFP: enhanced green fluorescent protein; EM: electron microscopy; ER: endoplasmic reticulum; FC: floxed control; FIS1: fission, mitochondrial 1; FUNDC1: FUN14 domain containing 1; H-E: hematoxylin-eosin; HIF1A: hypoxia inducible factor 1 subunit alpha; HSPD1: heat shock protein family D (Hsp60) member 1; IMMT/MIC60: inner membrane mitochondrial protein; IPC: ischemic preconditioning; I-R: ischemia-reperfusion; IRI: ischemia-reperfusion injury; JC-1: 5,5',6,6'-tetrachloro-1,1',3,3'-tetraethylbenzimidazolylcarbocyanine iodide; KO: knockout; MAP1LC3B/LC3B: microtubule associated protein 1 light chain 3 beta; mito-QC: mito-quality control; mRFP: monomeric red fluorescent protein; NAC: N-acetylcysteine; PINK1: PTEN induced putative kinase 1; PPIB: peptidylprolyl isomerase B; PRKN: parkin RBR E3 ubiquitin protein ligase; ROS: reactive oxygen species; RPTC: rat proximal tubular cells; SD: standard deviation; sIPC: simulated IPC; SQSTM1/p62: sequestosome 1; TOMM20: translocase of outer mitochondrial membrane 20; TUNEL: terminal deoxynucleotidyl transferase-mediated dUTP nick end labeling.
Keywords: Acute kidney injury; autophagy; ischemic preconditioning; mitophagy; proximal tubule; renal ischemia-reperfusion.
Figures
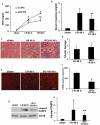
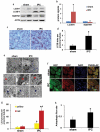
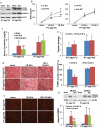
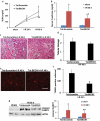
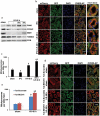
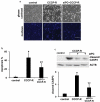
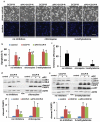
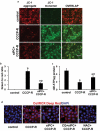
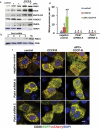
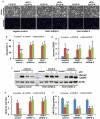
Similar articles
-
Mitochondria ROS and mitophagy in acute kidney injury.Autophagy. 2023 Feb;19(2):401-414. doi: 10.1080/15548627.2022.2084862. Epub 2022 Jun 9. Autophagy. 2023. PMID: 35678504 Free PMC article.
-
BNIP3L-mediated mitophagy is required for mitochondrial remodeling during the differentiation of optic nerve oligodendrocytes.Autophagy. 2021 Oct;17(10):3140-3159. doi: 10.1080/15548627.2020.1871204. Epub 2021 Jan 19. Autophagy. 2021. PMID: 33404293 Free PMC article.
-
BNIP3L/NIX degradation leads to mitophagy deficiency in ischemic brains.Autophagy. 2021 Aug;17(8):1934-1946. doi: 10.1080/15548627.2020.1802089. Epub 2020 Aug 12. Autophagy. 2021. PMID: 32722981 Free PMC article.
-
Organelle-specific autophagy in inflammatory diseases: a potential therapeutic target underlying the quality control of multiple organelles.Autophagy. 2021 Feb;17(2):385-401. doi: 10.1080/15548627.2020.1725377. Epub 2020 Feb 12. Autophagy. 2021. PMID: 32048886 Free PMC article. Review.
-
Impaired autophagy and APP processing in Alzheimer's disease: The potential role of Beclin 1 interactome.Prog Neurobiol. 2013 Jul-Aug;106-107:33-54. doi: 10.1016/j.pneurobio.2013.06.002. Epub 2013 Jul 1. Prog Neurobiol. 2013. PMID: 23827971 Review.
Cited by
-
LncRNA SNHG17 knockdown promotes Parkin-dependent mitophagy and reduces apoptosis of podocytes through Mst1.Cell Cycle. 2020 Aug;19(16):1997-2006. doi: 10.1080/15384101.2020.1783481. Epub 2020 Jul 5. Cell Cycle. 2020. PMID: 32627655 Free PMC article.
-
Comment on "mt-Keima detects PINK1-PRKN mitophagy in vivo with greater sensitivity than mito-QC".Autophagy. 2021 Dec;17(12):4477-4479. doi: 10.1080/15548627.2021.1907269. Epub 2021 Apr 5. Autophagy. 2021. PMID: 33818280 Free PMC article. No abstract available.
-
Lycium Barbarum polysaccharide protects HaCaT cells from PM2.5-induced apoptosis via inhibiting oxidative stress, ER stress and autophagy.Redox Rep. 2022 Dec;27(1):32-44. doi: 10.1080/13510002.2022.2036507. Redox Rep. 2022. PMID: 35130817 Free PMC article.
-
Tubular cells produce FGF2 via autophagy after acute kidney injury leading to fibroblast activation and renal fibrosis.Autophagy. 2023 Jan;19(1):256-277. doi: 10.1080/15548627.2022.2072054. Epub 2022 May 18. Autophagy. 2023. PMID: 35491858 Free PMC article.
-
Salvianolic acid B alleviates diabetic endothelial and mitochondrial dysfunction by down-regulating apoptosis and mitophagy of endothelial cells.Bioengineered. 2022 Feb;13(2):3486-3502. doi: 10.1080/21655979.2022.2026552. Bioengineered. 2022. PMID: 35068334 Free PMC article.
References
-
- Yellon DM, Downey JM.. Preconditioning the myocardium: from cellular physiology to clinical cardiology. Physiol Rev. 2003. October;83(4):1113–1151. . PubMed PMID: 14506302. - PubMed
-
- Pasupathy S, Homer-Vanniasinkam S. Ischaemic preconditioning protects against ischaemia/reperfusion injury: emerging concepts. Eur J Vasc Endovasc Surg. 2005. February;29(2):106–115. . PubMed PMID: 15649715. - PubMed
-
- Bonventre JV. Kidney ischemic preconditioning. Curr Opin Nephrol Hypertens. 2002. January;11(1):43–48. PubMed PMID: 11753086. - PubMed
Publication types
MeSH terms
Substances
Grants and funding
LinkOut - more resources
Full Text Sources
Other Literature Sources
Molecular Biology Databases
Research Materials
Miscellaneous