Polyploid Hepatocytes Facilitate Adaptation and Regeneration to Chronic Liver Injury
- PMID: 30928253
- PMCID: PMC6547059
- DOI: 10.1016/j.ajpath.2019.02.008
Polyploid Hepatocytes Facilitate Adaptation and Regeneration to Chronic Liver Injury
Abstract
The liver contains diploid and polyploid hepatocytes (tetraploid, octaploid, etc.), with polyploids comprising ≥90% of the hepatocyte population in adult mice. Polyploid hepatocytes form multipolar spindles in mitosis, which lead to chromosome gains/losses and random aneuploidy. The effect of aneuploidy on liver function is unclear, and the degree of liver aneuploidy is debated, with reports showing aneuploidy affects 5% to 60% of hepatocytes. To study relationships among liver polyploidy, aneuploidy, and adaptation, mice lacking E2f7 and E2f8 in the liver (LKO), which have a polyploidization defect, were used. Polyploids were reduced fourfold in LKO livers, and LKO hepatocytes remained predominantly diploid after extensive proliferation. Moreover, nearly all LKO hepatocytes were euploid compared with control hepatocytes, suggesting polyploid hepatocytes are required for production of aneuploid progeny. To determine whether reduced polyploidy impairs adaptation, LKO mice were bred onto a tyrosinemia background, a disease model whereby the liver can develop disease-resistant, regenerative nodules. Although tyrosinemic LKO mice were more susceptible to morbidities and death associated with tyrosinemia-induced liver failure, they developed regenerating nodules similar to control mice. Analyses revealed that nodules in the tyrosinemic livers were generated by aneuploidy and inactivating mutations. In summary, we identified new roles for polyploid hepatocytes and demonstrated that they are required for the formation of aneuploid progeny and can facilitate adaptation to chronic liver disease.
Copyright © 2019 American Society for Investigative Pathology. Published by Elsevier Inc. All rights reserved.
Figures
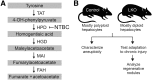
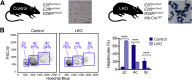
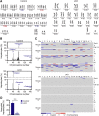
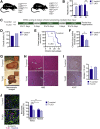
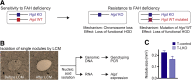
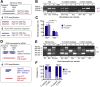
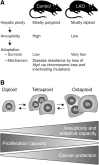
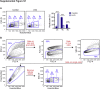
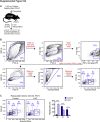
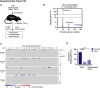
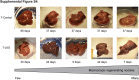
Similar articles
-
The Polyploid State Restricts Hepatocyte Proliferation and Liver Regeneration in Mice.Hepatology. 2019 Mar;69(3):1242-1258. doi: 10.1002/hep.30286. Epub 2019 Feb 15. Hepatology. 2019. PMID: 30244478 Free PMC article.
-
Aneuploidy, polyploidy and ploidy reversal in the liver.Semin Cell Dev Biol. 2013 Apr;24(4):347-56. doi: 10.1016/j.semcdb.2013.01.003. Epub 2013 Jan 16. Semin Cell Dev Biol. 2013. PMID: 23333793 Review.
-
Mice With Increased Numbers of Polyploid Hepatocytes Maintain Regenerative Capacity But Develop Fewer Hepatocellular Carcinomas Following Chronic Liver Injury.Gastroenterology. 2020 May;158(6):1698-1712.e14. doi: 10.1053/j.gastro.2020.01.026. Epub 2020 Jan 20. Gastroenterology. 2020. PMID: 31972235 Free PMC article.
-
The Polyploid State Plays a Tumor-Suppressive Role in the Liver.Dev Cell. 2018 Feb 26;44(4):447-459.e5. doi: 10.1016/j.devcel.2018.01.010. Epub 2018 Feb 8. Dev Cell. 2018. PMID: 29429824 Free PMC article.
-
Differential Roles for Diploid and Polyploid Hepatocytes in Acute and Chronic Liver Injury.Semin Liver Dis. 2021 Jan;41(1):42-49. doi: 10.1055/s-0040-1719175. Epub 2020 Dec 14. Semin Liver Dis. 2021. PMID: 33764484 Free PMC article. Review.
Cited by
-
Ploidy dynamics increase the risk of liver cancer initiation.Nat Commun. 2021 Mar 25;12(1):1896. doi: 10.1038/s41467-021-21897-8. Nat Commun. 2021. PMID: 33767143 Free PMC article.
-
Cellular polyploidy in organ homeostasis and regeneration.Protein Cell. 2023 Aug 1;14(8):560-578. doi: 10.1093/procel/pwac064. Protein Cell. 2023. PMID: 37526344 Free PMC article.
-
Raising of Anillin expression in para-cancerous hepatocytes is associated with hepatic depolyploidization and short-term recurrence of hepatocellular carcinoma after radical operation.J Cancer. 2022 May 29;13(9):2729-2739. doi: 10.7150/jca.72890. eCollection 2022. J Cancer. 2022. PMID: 35812180 Free PMC article.
-
Adult Cardiomyocyte Cell Cycle Detour: Off-ramp to Quiescent Destinations.Trends Endocrinol Metab. 2019 Aug;30(8):557-567. doi: 10.1016/j.tem.2019.05.006. Epub 2019 Jun 28. Trends Endocrinol Metab. 2019. PMID: 31262545 Free PMC article. Review.
-
Hepatocyte Polyploidy: Driver or Gatekeeper of Chronic Liver Diseases.Cancers (Basel). 2021 Oct 14;13(20):5151. doi: 10.3390/cancers13205151. Cancers (Basel). 2021. PMID: 34680300 Free PMC article. Review.
References
-
- Gentric G., Desdouets C. Polyploidization in liver tissue. Am J Pathol. 2014;184:322–331. - PubMed
-
- Pandit S.K., Westendorp B., de Bruin A. Physiological significance of polyploidization in mammalian cells. Trends Cell Biol. 2013;23:556–566. - PubMed
-
- Duncan A.W. Aneuploidy, polyploidy and ploidy reversal in the liver. Semin Cell Dev Biol. 2013;24:347–356. - PubMed
-
- Margall-Ducos G., Celton-Morizur S., Couton D., Bregerie O., Desdouets C. Liver tetraploidization is controlled by a new process of incomplete cytokinesis. J Cell Sci. 2007;120:3633–3639. - PubMed
Publication types
MeSH terms
Substances
Grants and funding
LinkOut - more resources
Full Text Sources
Molecular Biology Databases