Gboxin is an oxidative phosphorylation inhibitor that targets glioblastoma
- PMID: 30842654
- PMCID: PMC6655586
- DOI: 10.1038/s41586-019-0993-x
Gboxin is an oxidative phosphorylation inhibitor that targets glioblastoma
Abstract
Cancer-specific inhibitors that reflect the unique metabolic needs of cancer cells are rare. Here we describe Gboxin, a small molecule that specifically inhibits the growth of primary mouse and human glioblastoma cells but not that of mouse embryonic fibroblasts or neonatal astrocytes. Gboxin rapidly and irreversibly compromises oxygen consumption in glioblastoma cells. Gboxin relies on its positive charge to associate with mitochondrial oxidative phosphorylation complexes in a manner that is dependent on the proton gradient of the inner mitochondrial membrane, and it inhibits the activity of F0F1 ATP synthase. Gboxin-resistant cells require a functional mitochondrial permeability transition pore that regulates pH and thus impedes the accumulation of Gboxin in the mitochondrial matrix. Administration of a metabolically stable Gboxin analogue inhibits glioblastoma allografts and patient-derived xenografts. Gboxin toxicity extends to established human cancer cell lines of diverse organ origin, and shows that the increased proton gradient and pH in cancer cell mitochondria is a mode of action that can be targeted in the development of antitumour reagents.
Figures
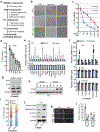
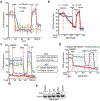
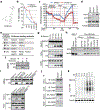
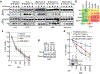
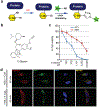
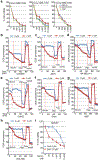
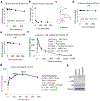
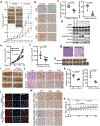
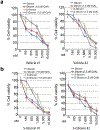
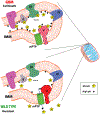
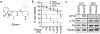
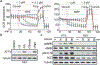
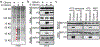
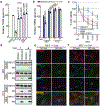
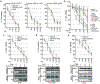
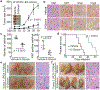
Comment in
-
Targeting cancer cell metabolism in glioblastoma.Nat Rev Cancer. 2019 May;19(5):250. doi: 10.1038/s41568-019-0139-3. Nat Rev Cancer. 2019. PMID: 30944412 No abstract available.
Similar articles
-
Cancer cell-mitochondria hybrid membrane coated Gboxin loaded nanomedicines for glioblastoma treatment.Nat Commun. 2023 Jul 28;14(1):4557. doi: 10.1038/s41467-023-40280-3. Nat Commun. 2023. PMID: 37507371 Free PMC article.
-
Glycogen synthase kinase 3 inhibition slows mitochondrial adenine nucleotide transport and regulates voltage-dependent anion channel phosphorylation.Circ Res. 2008 Oct 24;103(9):983-91. doi: 10.1161/CIRCRESAHA.108.178970. Epub 2008 Sep 18. Circ Res. 2008. PMID: 18802025 Free PMC article.
-
Lateral pH gradient between OXPHOS complex IV and F(0)F(1) ATP-synthase in folded mitochondrial membranes.Nat Commun. 2014;5:3103. doi: 10.1038/ncomms4103. Nat Commun. 2014. PMID: 24476986
-
Modulation of the mitochondrial permeability transition by cyclophilin D: moving closer to F(0)-F(1) ATP synthase?Mitochondrion. 2012 Jan;12(1):41-5. doi: 10.1016/j.mito.2011.04.007. Epub 2011 May 8. Mitochondrion. 2012. PMID: 21586346 Review.
-
Genetic control of oxidative phosphorylation and experimental models of defects.Hum Reprod. 2000 Jul;15 Suppl 2:18-27. doi: 10.1093/humrep/15.suppl_2.18. Hum Reprod. 2000. PMID: 11041510 Review.
Cited by
-
Disrupting metformin adaptation of liver cancer cells by targeting the TOMM34/ATP5B axis.EMBO Mol Med. 2022 Dec 7;14(12):e16082. doi: 10.15252/emmm.202216082. Epub 2022 Nov 2. EMBO Mol Med. 2022. PMID: 36321555 Free PMC article.
-
Selective cytotoxicity of a novel mitochondrial complex I inhibitor, YK-135, against EMT-subtype gastric cancer cell lines due to impaired glycolytic capacity.BMB Rep. 2022 Dec;55(12):645-650. doi: 10.5483/BMBRep.2022.55.12.150. BMB Rep. 2022. PMID: 36379512 Free PMC article.
-
Dual-locked fluorescent probes for precise diagnosis and targeted treatment of tumors.Heliyon. 2024 Sep 19;10(18):e38174. doi: 10.1016/j.heliyon.2024.e38174. eCollection 2024 Sep 30. Heliyon. 2024. PMID: 39381214 Free PMC article. Review.
-
Stellettin B Sensitizes Glioblastoma to DNA-Damaging Treatments by Suppressing PI3K-Mediated Homologous Recombination Repair.Adv Sci (Weinh). 2023 Jan;10(3):e2205529. doi: 10.1002/advs.202205529. Epub 2022 Dec 1. Adv Sci (Weinh). 2023. PMID: 36453577 Free PMC article.
-
Mitochondrial Metabolism as a Target for Cancer Therapy.Cell Metab. 2020 Sep 1;32(3):341-352. doi: 10.1016/j.cmet.2020.06.019. Epub 2020 Jul 14. Cell Metab. 2020. PMID: 32668195 Free PMC article. Review.
References
MeSH terms
Substances
Grants and funding
LinkOut - more resources
Full Text Sources
Other Literature Sources
Molecular Biology Databases