Modeling Herpes Simplex Virus 1 Infections in Human Central Nervous System Neuronal Cells Using Two- and Three-Dimensional Cultures Derived from Induced Pluripotent Stem Cells
- PMID: 30787148
- PMCID: PMC6475775
- DOI: 10.1128/JVI.00111-19
Modeling Herpes Simplex Virus 1 Infections in Human Central Nervous System Neuronal Cells Using Two- and Three-Dimensional Cultures Derived from Induced Pluripotent Stem Cells
Abstract
Herpes simplex virus 1 (HSV-1) establishes latency in both peripheral nerve ganglia and the central nervous system (CNS). The outcomes of acute and latent infections in these different anatomic sites appear to be distinct. It is becoming clear that many of the existing culture models using animal primary neurons to investigate HSV-1 infection of the CNS are limited and not ideal, and most do not recapitulate features of CNS neurons. Human induced pluripotent stem cells (hiPSCs) and neurons derived from them are documented as tools to study aspects of neuropathogenesis, but few have focused on modeling infections of the CNS. Here, we characterize functional two-dimensional (2D) CNS-like neuron cultures and three-dimensional (3D) brain organoids made from hiPSCs to model HSV-1-human-CNS interactions. Our results show that (i) hiPSC-derived CNS neurons are permissive for HSV-1 infection; (ii) a quiescent state exhibiting key landmarks of HSV-1 latency described in animal models can be established in hiPSC-derived CNS neurons; (iii) the complex laminar structure of the organoids can be efficiently infected with HSV, with virus being transported from the periphery to the central layers of the organoid; and (iv) the organoids support reactivation of HSV-1, albeit less efficiently than 2D cultures. Collectively, our results indicate that hiPSC-derived neuronal platforms, especially 3D organoids, offer an extraordinary opportunity for modeling the interaction of HSV-1 with the complex cellular and architectural structure of the human CNS.IMPORTANCE This study employed human induced pluripotent stem cells (hiPSCs) to model acute and latent HSV-1 infections in two-dimensional (2D) and three-dimensional (3D) CNS neuronal cultures. We successfully established acute HSV-1 infections and infections showing features of latency. HSV-1 infection of the 3D organoids was able to spread from the outer surface of the organoid and was transported to the interior lamina, providing a model to study HSV-1 trafficking through complex neuronal tissue structures. HSV-1 could be reactivated in both culture systems; though, in contrast to 2D cultures, it appeared to be more difficult to reactivate HSV-1 in 3D cultures, potentially paralleling the low efficiency of HSV-1 reactivation in the CNS of animal models. The reactivation events were accompanied by dramatic neuronal morphological changes and cell-cell fusion. Together, our results provide substantive evidence of the suitability of hiPSC-based neuronal platforms to model HSV-1-CNS interactions in a human context.
Keywords: herpes simplex virus 1 (HSV-1); human induced pluripotent stem cells (hiPSCs); neurodegeneration; organoid; three-dimensional (3D) neuronal cultures.
Copyright © 2019 D’Aiuto et al.
Figures
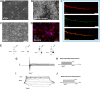
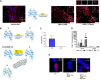
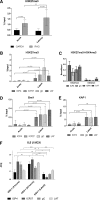
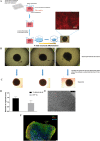
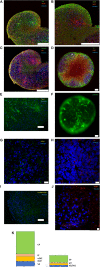
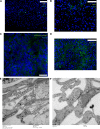
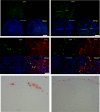
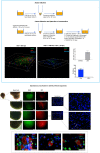
Similar articles
-
Patterns of Herpes Simplex Virus 1 Infection in Neural Progenitor Cells.J Virol. 2020 Jul 30;94(16):e00994-20. doi: 10.1128/JVI.00994-20. Print 2020 Jul 30. J Virol. 2020. PMID: 32493817 Free PMC article.
-
Herpes Simplex Virus 1 Strains 17syn+ and KOS(M) Differ Greatly in Their Ability To Reactivate from Human Neurons In Vitro.J Virol. 2020 Jul 16;94(15):e00796-20. doi: 10.1128/JVI.00796-20. Print 2020 Jul 16. J Virol. 2020. PMID: 32461310 Free PMC article.
-
Infectious Herpes Simplex Virus in the Brain Stem Is Correlated with Reactivation in the Trigeminal Ganglia.J Virol. 2019 Apr 3;93(8):e02209-18. doi: 10.1128/JVI.02209-18. Print 2019 Apr 15. J Virol. 2019. PMID: 30728262 Free PMC article.
-
Animal models of herpes simplex virus immunity and pathogenesis.J Neurovirol. 2015 Feb;21(1):8-23. doi: 10.1007/s13365-014-0302-2. Epub 2014 Nov 12. J Neurovirol. 2015. PMID: 25388226 Review.
-
Defining nervous system susceptibility during acute and latent herpes simplex virus-1 infection.J Neuroimmunol. 2017 Jul 15;308:43-49. doi: 10.1016/j.jneuroim.2017.02.020. Epub 2017 Mar 8. J Neuroimmunol. 2017. PMID: 28302316 Free PMC article. Review.
Cited by
-
iPSC-derived three-dimensional brain organoid models and neurotropic viral infections.J Neurovirol. 2023 Apr;29(2):121-134. doi: 10.1007/s13365-023-01133-3. Epub 2023 Apr 25. J Neurovirol. 2023. PMID: 37097597 Free PMC article. Review.
-
3D engineered tissue models for studying human-specific infectious viral diseases.Bioact Mater. 2022 Sep 22;21:576-594. doi: 10.1016/j.bioactmat.2022.09.010. eCollection 2023 Mar. Bioact Mater. 2022. PMID: 36204281 Free PMC article. Review.
-
Advancing Drug Discovery for Neurological Disorders Using iPSC-Derived Neural Organoids.Int J Mol Sci. 2021 Mar 6;22(5):2659. doi: 10.3390/ijms22052659. Int J Mol Sci. 2021. PMID: 33800815 Free PMC article. Review.
-
The HSV-1 ubiquitin ligase ICP0: Modifying the cellular proteome to promote infection.Virus Res. 2020 Aug;285:198015. doi: 10.1016/j.virusres.2020.198015. Epub 2020 May 13. Virus Res. 2020. PMID: 32416261 Free PMC article. Review.
-
Contribution of CNS and extra-CNS infections to neurodegeneration: a narrative review.J Neuroinflammation. 2024 Jun 6;21(1):152. doi: 10.1186/s12974-024-03139-y. J Neuroinflammation. 2024. PMID: 38845026 Free PMC article. Review.
References
MeSH terms
Grants and funding
LinkOut - more resources
Full Text Sources
Medical