In Situ Bioprinting of Autologous Skin Cells Accelerates Wound Healing of Extensive Excisional Full-Thickness Wounds
- PMID: 30755653
- PMCID: PMC6372693
- DOI: 10.1038/s41598-018-38366-w
In Situ Bioprinting of Autologous Skin Cells Accelerates Wound Healing of Extensive Excisional Full-Thickness Wounds
Abstract
The early treatment and rapid closure of acute or chronic wounds is essential for normal healing and prevention of hypertrophic scarring. The use of split thickness autografts is often limited by the availability of a suitable area of healthy donor skin to harvest. Cellular and non-cellular biological skin-equivalents are commonly used as an alternative treatment option for these patients, however these treatments usually involve multiple surgical procedures and associated with high costs of production and repeated wound treatment. Here we describe a novel design and a proof-of-concept validation of a mobile skin bioprinting system that provides rapid on-site management of extensive wounds. Integrated imaging technology facilitated the precise delivery of either autologous or allogeneic dermal fibroblasts and epidermal keratinocytes directly into an injured area, replicating the layered skin structure. Excisional wounds bioprinted with layered autologous dermal fibroblasts and epidermal keratinocytes in a hydrogel carrier showed rapid wound closure, reduced contraction and accelerated re-epithelialization. These regenerated tissues had a dermal structure and composition similar to healthy skin, with extensive collagen deposition arranged in large, organized fibers, extensive mature vascular formation and proliferating keratinocytes.
Conflict of interest statement
The authors declare no competing interests.
Figures
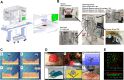
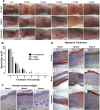
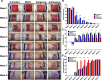
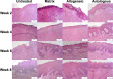
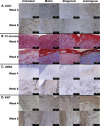
Similar articles
-
Bioprinted Skin Recapitulates Normal Collagen Remodeling in Full-Thickness Wounds.Tissue Eng Part A. 2020 May;26(9-10):512-526. doi: 10.1089/ten.TEA.2019.0319. Epub 2020 Jan 28. Tissue Eng Part A. 2020. PMID: 31861970 Free PMC article.
-
Rapid creation of skin substitutes from human skin cells and biomimetic nanofibers for acute full-thickness wound repair.Burns. 2015 Dec;41(8):1764-1774. doi: 10.1016/j.burns.2015.06.011. Epub 2015 Jul 15. Burns. 2015. PMID: 26187057 Free PMC article.
-
Solubilized Amnion Membrane Hyaluronic Acid Hydrogel Accelerates Full-Thickness Wound Healing.Stem Cells Transl Med. 2017 Nov;6(11):2020-2032. doi: 10.1002/sctm.17-0053. Epub 2017 Sep 23. Stem Cells Transl Med. 2017. PMID: 28941321 Free PMC article.
-
Tissue-engineered skin. Current status in wound healing.Am J Clin Dermatol. 2001;2(5):305-13. doi: 10.2165/00128071-200102050-00005. Am J Clin Dermatol. 2001. PMID: 11721649 Review.
-
Recent Advances in the Design of Three-Dimensional and Bioprinted Scaffolds for Full-Thickness Wound Healing.Tissue Eng Part B Rev. 2022 Feb;28(1):160-181. doi: 10.1089/ten.TEB.2020.0339. Epub 2021 Feb 22. Tissue Eng Part B Rev. 2022. PMID: 33446047 Review.
Cited by
-
Current Advances of Three-Dimensional Bioprinting Application in Dentistry: A Scoping Review.Materials (Basel). 2022 Sep 15;15(18):6398. doi: 10.3390/ma15186398. Materials (Basel). 2022. PMID: 36143709 Free PMC article. Review.
-
Advancing cardiac regeneration through 3D bioprinting: methods, applications, and future directions.Heart Fail Rev. 2024 May;29(3):599-613. doi: 10.1007/s10741-023-10367-6. Epub 2023 Nov 9. Heart Fail Rev. 2024. PMID: 37943420 Review.
-
Bioprinted Skin Recapitulates Normal Collagen Remodeling in Full-Thickness Wounds.Tissue Eng Part A. 2020 May;26(9-10):512-526. doi: 10.1089/ten.TEA.2019.0319. Epub 2020 Jan 28. Tissue Eng Part A. 2020. PMID: 31861970 Free PMC article.
-
Robotic-assisted automated in situ bioprinting.Int J Bioprint. 2022 Oct 28;9(1):629. doi: 10.18063/ijb.v9i1.629. eCollection 2023. Int J Bioprint. 2022. PMID: 36636132 Free PMC article. Review.
-
3D Printing and Virtual Surgical Planning in Oral and Maxillofacial Surgery.J Clin Med. 2022 Apr 24;11(9):2385. doi: 10.3390/jcm11092385. J Clin Med. 2022. PMID: 35566511 Free PMC article. Review.
References
-
- Beckrich K, Aronovitch SA. Hospital-acquired pressure ulcers: a comparison of costs in medical vs. surgical patients. Nurs Econ. 1999;17:263–271. - PubMed
-
- Miller, S. F. et al. National burn repository 2007 report: a synopsis of the 2007 call for data. J Burn Care Res29, 862–870, discussion 871 (2008). - PubMed
-
- Thomas SJ, Kramer GC, Herndon DN. Burns: military options and tactical solutions. J Trauma. 2003;54:S207–218. - PubMed
Publication types
MeSH terms
Substances
LinkOut - more resources
Full Text Sources
Other Literature Sources