Drosophila as a Genetic Model for Hematopoiesis
- PMID: 30733377
- PMCID: PMC6366919
- DOI: 10.1534/genetics.118.300223
Drosophila as a Genetic Model for Hematopoiesis
Abstract
In this FlyBook chapter, we present a survey of the current literature on the development of the hematopoietic system in Drosophila The Drosophila blood system consists entirely of cells that function in innate immunity, tissue integrity, wound healing, and various forms of stress response, and are therefore functionally similar to myeloid cells in mammals. The primary cell types are specialized for phagocytic, melanization, and encapsulation functions. As in mammalian systems, multiple sites of hematopoiesis are evident in Drosophila and the mechanisms involved in this process employ many of the same molecular strategies that exemplify blood development in humans. Drosophila blood progenitors respond to internal and external stress by coopting developmental pathways that involve both local and systemic signals. An important goal of these Drosophila studies is to develop the tools and mechanisms critical to further our understanding of human hematopoiesis during homeostasis and dysfunction.
Keywords: Drosophila; FlyBook; crystal cell; hematopoiesis; hemocyte; innate immunity; lamellocyte; lymph gland; plasmatocyte; stress response.
Copyright © 2019 Banerjee et al.
Figures
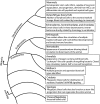
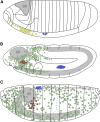
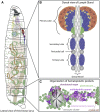
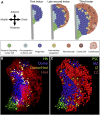
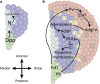
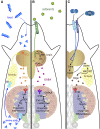
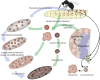
Similar articles
-
Drosophila as a Model to Study Cellular Communication Between the Hematopoietic Niche and Blood Progenitors Under Homeostatic Conditions and in Response to an Immune Stress.Front Immunol. 2021 Aug 16;12:719349. doi: 10.3389/fimmu.2021.719349. eCollection 2021. Front Immunol. 2021. PMID: 34484226 Free PMC article. Review.
-
Hematopoietic progenitors and hemocyte lineages in the Drosophila lymph gland.Dev Biol. 2010 Oct 15;346(2):310-9. doi: 10.1016/j.ydbio.2010.08.003. Epub 2010 Aug 10. Dev Biol. 2010. PMID: 20707995
-
The Drosophila lymph gland is an ideal model for studying hematopoiesis.Dev Comp Immunol. 2018 Jun;83:60-69. doi: 10.1016/j.dci.2017.11.017. Epub 2017 Nov 27. Dev Comp Immunol. 2018. PMID: 29191551 Review.
-
Intrinsic and Extrinsic Regulation of Hematopoiesis in Drosophila.Mol Cells. 2022 Mar 31;45(3):101-108. doi: 10.14348/molcells.2022.2039. Mol Cells. 2022. PMID: 35253654 Free PMC article. Review.
-
Ontogeny of the Drosophila larval hematopoietic organ, hemocyte homeostasis and the dedicated cellular immune response to parasitism.Int J Dev Biol. 2010;54(6-7):1117-25. doi: 10.1387/ijdb.093053jk. Int J Dev Biol. 2010. PMID: 20711989 Review.
Cited by
-
The role of micro RNAs (miRNAs) in the regulation of Drosophila melanogaster's innate immunity.Fly (Austin). 2022 Dec;16(1):382-396. doi: 10.1080/19336934.2022.2149204. Fly (Austin). 2022. PMID: 36412256 Free PMC article. Review.
-
Dysregulation of innate immune signaling in animal models of Spinal Muscular Atrophy.bioRxiv [Preprint]. 2023 Dec 15:2023.12.14.571739. doi: 10.1101/2023.12.14.571739. bioRxiv. 2023. Update in: BMC Biol. 2024 Apr 25;22(1):94. doi: 10.1186/s12915-024-01888-z. PMID: 38168196 Free PMC article. Updated. Preprint.
-
Identification of Bipotential Blood Cell/Nephrocyte Progenitors in Drosophila: Another Route for Generating Blood Progenitors.Front Cell Dev Biol. 2022 Feb 14;10:834720. doi: 10.3389/fcell.2022.834720. eCollection 2022. Front Cell Dev Biol. 2022. PMID: 35237606 Free PMC article.
-
Intact in situ Preparation of the Drosophila melanogaster Lymph Gland for a Comprehensive Analysis of Larval Hematopoiesis.Bio Protoc. 2021 Nov 5;11(21):e4204. doi: 10.21769/BioProtoc.4204. eCollection 2021 Nov 5. Bio Protoc. 2021. PMID: 34859119 Free PMC article.
-
Chk2-p53 and JNK in irradiation-induced cell death of hematopoietic progenitors and differentiated cells in Drosophila larval lymph gland.Biol Open. 2021 Aug 15;10(8):bio058809. doi: 10.1242/bio.058809. Epub 2021 Aug 23. Biol Open. 2021. PMID: 34328173 Free PMC article.
References
-
- Abel T., Michelson A. M., Maniatis T., 1993. A Drosophila GATA family member that binds to Adh regulatory sequences is expressed in the developing fat body. Development 119: 623–633. - PubMed
Publication types
MeSH terms
Grants and funding
LinkOut - more resources
Full Text Sources
Molecular Biology Databases
Research Materials