Double-Stranded RNA Sensors and Modulators in Innate Immunity
- PMID: 30673536
- PMCID: PMC7136661
- DOI: 10.1146/annurev-immunol-042718-041356
Double-Stranded RNA Sensors and Modulators in Innate Immunity
Abstract
Detection of double-stranded RNAs (dsRNAs) is a central mechanism of innate immune defense in many organisms. We here discuss several families of dsRNA-binding proteins involved in mammalian antiviral innate immunity. These include RIG-I-like receptors, protein kinase R, oligoadenylate synthases, adenosine deaminases acting on RNA, RNA interference systems, and other proteins containing dsRNA-binding domains and helicase domains. Studies suggest that their functions are highly interdependent and that their interdependence could offer keys to understanding the complex regulatory mechanisms for cellular dsRNA homeostasis and antiviral immunity. This review aims to highlight their interconnectivity, as well as their commonalities and differences in their dsRNA recognition mechanisms.
Keywords: RIG-I-like receptor; RNA interference; dsRNA; dsRNA-dependent adenosine deaminase; oligoadenylate synthase; protein kinase R.
Figures
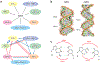
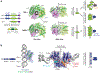
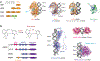
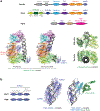
Similar articles
-
Activation of innate immunity by mitochondrial dsRNA in mouse cells lacking p53 protein.RNA. 2019 Jun;25(6):713-726. doi: 10.1261/rna.069625.118. Epub 2019 Mar 20. RNA. 2019. PMID: 30894411 Free PMC article.
-
Structural and functional analysis reveals that human OASL binds dsRNA to enhance RIG-I signaling.Nucleic Acids Res. 2015 May 26;43(10):5236-48. doi: 10.1093/nar/gkv389. Epub 2015 Apr 29. Nucleic Acids Res. 2015. PMID: 25925578 Free PMC article.
-
Molecular basis for an attenuated cytoplasmic dsRNA response in human embryonic stem cells.Cell Cycle. 2010 Sep 1;9(17):3552-64. doi: 10.4161/cc.9.17.12792. Epub 2010 Sep 24. Cell Cycle. 2010. PMID: 20814227 Free PMC article.
-
What Really Rigs Up RIG-I?J Innate Immun. 2016;8(5):429-36. doi: 10.1159/000447947. Epub 2016 Jul 21. J Innate Immun. 2016. PMID: 27438016 Free PMC article. Review.
-
Structures of RIG-I-Like Receptors and Insights into Viral RNA Sensing.Adv Exp Med Biol. 2019;1172:157-188. doi: 10.1007/978-981-13-9367-9_8. Adv Exp Med Biol. 2019. PMID: 31628656 Review.
Cited by
-
Picornaviruses: A View from 3A.Viruses. 2021 Mar 11;13(3):456. doi: 10.3390/v13030456. Viruses. 2021. PMID: 33799649 Free PMC article. Review.
-
SARS-CoV-2 biology and variants: anticipation of viral evolution and what needs to be done.Environ Microbiol. 2021 May;23(5):2339-2363. doi: 10.1111/1462-2920.15487. Epub 2021 Apr 5. Environ Microbiol. 2021. PMID: 33769683 Free PMC article.
-
Coronavirus: a shift in focus away from IFN response and towards other inflammatory targets.J Cell Commun Signal. 2020 Dec;14(4):469-470. doi: 10.1007/s12079-020-00574-3. Epub 2020 Sep 7. J Cell Commun Signal. 2020. PMID: 32895779 Free PMC article.
-
Deep learning models for predicting RNA degradation via dual crowdsourcing.Nat Mach Intell. 2022;4(12):1174-1184. doi: 10.1038/s42256-022-00571-8. Epub 2022 Dec 14. Nat Mach Intell. 2022. PMID: 36567960 Free PMC article.
-
Transcriptome-wide changes in gene expression, splicing, and lncRNAs in response to a live attenuated dengue virus vaccine.Cell Rep. 2022 Feb 8;38(6):110341. doi: 10.1016/j.celrep.2022.110341. Cell Rep. 2022. PMID: 35139383 Free PMC article.
References
-
- Neidle S 2008. Principles of Nucleic Acid Structure. London: Academic
-
- Gesteland RF, Cech TR, Atkins JF. 1999. The RNA World. Cold Spring Harbor, NY: Cold Spring Harb. Lab. Press; 2nd ed.
Publication types
MeSH terms
Substances
Grants and funding
LinkOut - more resources
Full Text Sources
Other Literature Sources
Medical
Research Materials