A minimal biochemical route towards de novo formation of synthetic phospholipid membranes
- PMID: 30655537
- PMCID: PMC6336818
- DOI: 10.1038/s41467-018-08174-x
A minimal biochemical route towards de novo formation of synthetic phospholipid membranes
Abstract
All living cells consist of membrane compartments, which are mainly composed of phospholipids. Phospholipid synthesis is catalyzed by membrane-bound enzymes, which themselves require pre-existing membranes for function. Thus, the principle of membrane continuity creates a paradox when considering how the first biochemical membrane-synthesis machinery arose and has hampered efforts to develop simplified pathways for membrane generation in synthetic cells. Here, we develop a high-yielding strategy for de novo formation and growth of phospholipid membranes by repurposing a soluble enzyme FadD10 to form fatty acyl adenylates that react with amine-functionalized lysolipids to form phospholipids. Continuous supply of fresh precursors needed for lipid synthesis enables the growth of vesicles encapsulating FadD10. Using a minimal transcription/translation system, phospholipid vesicles are generated de novo in the presence of DNA encoding FadD10. Our findings suggest that alternate chemistries can produce and maintain synthetic phospholipid membranes and provides a strategy for generating membrane-based materials.
Conflict of interest statement
The authors declare no competing interests.
Figures
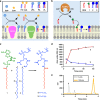
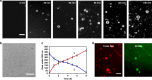
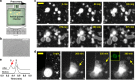
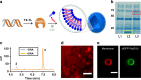
Similar articles
-
Expression of Fatty Acyl-CoA Ligase Drives One-Pot De Novo Synthesis of Membrane-Bound Vesicles in a Cell-Free Transcription-Translation System.J Am Chem Soc. 2021 Jul 28;143(29):11235-11242. doi: 10.1021/jacs.1c05394. Epub 2021 Jul 14. J Am Chem Soc. 2021. PMID: 34260248
-
Self-reproducing catalyst drives repeated phospholipid synthesis and membrane growth.Proc Natl Acad Sci U S A. 2015 Jul 7;112(27):8187-92. doi: 10.1073/pnas.1506704112. Epub 2015 Jun 22. Proc Natl Acad Sci U S A. 2015. PMID: 26100914 Free PMC article.
-
Chemoenzymatic Generation of Phospholipid Membranes Mediated by Type I Fatty Acid Synthase.J Am Chem Soc. 2021 Jun 16;143(23):8533-8537. doi: 10.1021/jacs.1c02121. Epub 2021 May 12. J Am Chem Soc. 2021. PMID: 33978402
-
Polyunsaturated fatty acid-phospholipid remodeling and inflammation.Curr Opin Endocrinol Diabetes Obes. 2015 Apr;22(2):112-8. doi: 10.1097/MED.0000000000000138. Curr Opin Endocrinol Diabetes Obes. 2015. PMID: 25692925 Review.
-
Metabolism of platelet activating factor and related ether lipids: enzymatic pathways, subcellular sites, regulation, and membrane processing.Prog Clin Biol Res. 1988;282:57-72. Prog Clin Biol Res. 1988. PMID: 3071807 Review.
Cited by
-
Cell-Free Protein Synthesis: Chassis toward the Minimal Cell.Cells. 2019 Apr 5;8(4):315. doi: 10.3390/cells8040315. Cells. 2019. PMID: 30959805 Free PMC article. Review.
-
Bioconjugation Strategies for Revealing the Roles of Lipids in Living Cells.Acc Chem Res. 2022 Nov 1;55(21):3099-3109. doi: 10.1021/acs.accounts.2c00511. Epub 2022 Oct 10. Acc Chem Res. 2022. PMID: 36215688 Free PMC article.
-
In situ formation of a biomimetic lipid membrane triggered by an aggregation-enhanced photoligation chemistry.Chem Sci. 2021 Jan 21;12(10):3627-3632. doi: 10.1039/d0sc06049f. Chem Sci. 2021. PMID: 34163636 Free PMC article.
-
De novo synthesized Min proteins drive oscillatory liposome deformation and regulate FtsA-FtsZ cytoskeletal patterns.Nat Commun. 2019 Oct 31;10(1):4969. doi: 10.1038/s41467-019-12932-w. Nat Commun. 2019. PMID: 31672986 Free PMC article.
-
On biochemical constructors and synthetic cells.Interface Focus. 2023 Aug 11;13(5):20230014. doi: 10.1098/rsfs.2023.0014. eCollection 2023 Oct 6. Interface Focus. 2023. PMID: 37577005 Free PMC article.
References
Publication types
MeSH terms
Substances
LinkOut - more resources
Full Text Sources
Other Literature Sources