Inositol monophosphatase 1 as a novel interacting partner of RAGE in pulmonary hypertension
- PMID: 30604625
- PMCID: PMC6425619
- DOI: 10.1152/ajplung.00393.2018
Inositol monophosphatase 1 as a novel interacting partner of RAGE in pulmonary hypertension
Abstract
Pulmonary arterial hypertension (PAH) is a lethal disease characterized by progressive pulmonary vascular remodeling. The receptor for advanced glycation end products (RAGE) plays an important role in PAH by promoting proliferation of pulmonary vascular cells. RAGE is also known to mediate activation of Akt signaling, although the particular molecular mechanism remains unknown. This study aimed to identify the interacting partner of RAGE that could facilitate RAGE-mediated Akt activation and vascular remodeling in PAH. The progressive angioproliferative PAH was induced in 24 female Sprague-Dawley rats ( n = 8/group) that were randomly assigned to develop PAH for 1, 2, or 5 wk [right ventricle systolic pressure (RVSP) 56.5 ± 3.2, 63.6 ± 1.6, and 111.1 ± 4.5 mmHg, respectively, vs. 22.9 ± 1.1 mmHg in controls]. PAH triggered early and late episodes of apoptosis in rat lungs accompanied by RAGE activation. Mass spectrometry analysis has identified IMPA1 as a novel PAH-specific interacting partner of RAGE. The proximity ligation assay (PLA) confirmed the formation of RAGE/IMPA1 complex in the pulmonary artery wall. Activation of IMPA1 in response to increased glucose 6-phosphate (G6P) is known to play a critical role in inositol synthesis and recycling. Indeed, we confirmed a threefold increase in G6P ( P = 0.0005) levels in lungs of PAH rats starting from week 1 that correlated with accumulation of phosphatidylinositol (3,4,5)-trisphosphate (PIP3), membrane translocation of PI3K, and a threefold increase in membrane Akt levels ( P = 0.02) and Akt phosphorylation. We conclude that the formation of the newly discovered RAGE-IMPA1 complex could be responsible for the stimulation of inositol pathways and activation of Akt signaling in PAH.
Keywords: glycolysis; inositol pathway; proliferation; pulmonary hypertension; receptor for advanced glycation end products.
Conflict of interest statement
No conflicts of interest, financial or otherwise, are declared by the authors.
Figures
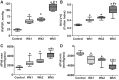
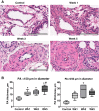
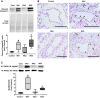
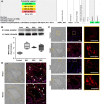
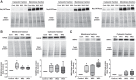
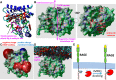
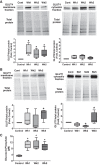
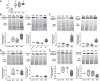
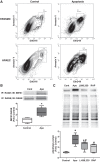
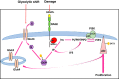
Similar articles
-
Critical role for the advanced glycation end-products receptor in pulmonary arterial hypertension etiology.J Am Heart Assoc. 2013 Jan 16;2(1):e005157. doi: 10.1161/JAHA.112.005157. J Am Heart Assoc. 2013. PMID: 23525442 Free PMC article.
-
RAGE-mediated extracellular matrix proteins accumulation exacerbates HySu-induced pulmonary hypertension.Cardiovasc Res. 2017 May 1;113(6):586-597. doi: 10.1093/cvr/cvx051. Cardiovasc Res. 2017. PMID: 28407046
-
Development of pulmonary arterial hypertension in mice over-expressing S100A4/Mts1 is specific to females.Respir Res. 2011 Dec 20;12(1):159. doi: 10.1186/1465-9921-12-159. Respir Res. 2011. PMID: 22185646 Free PMC article.
-
Potential of the TRPM7 channel as a novel therapeutic target for pulmonary arterial hypertension.J Smooth Muscle Res. 2022;58(0):50-62. doi: 10.1540/jsmr.58.50. J Smooth Muscle Res. 2022. PMID: 35944979 Free PMC article. Review.
-
The metabolic theory of pulmonary arterial hypertension.Circ Res. 2014 Jun 20;115(1):148-64. doi: 10.1161/CIRCRESAHA.115.301130. Circ Res. 2014. PMID: 24951764 Review.
Cited by
-
Mitochondria as a primary determinant of angiogenic modality in pulmonary arterial hypertension.J Exp Med. 2024 Nov 4;221(11):e20231568. doi: 10.1084/jem.20231568. Epub 2024 Sep 25. J Exp Med. 2024. PMID: 39320470
-
Exploring the therapeutic mechanisms of Coptidis Rhizoma in gastric precancerous lesions: a network pharmacology approach.Discov Oncol. 2024 Jun 5;15(1):211. doi: 10.1007/s12672-024-01070-5. Discov Oncol. 2024. PMID: 38837097 Free PMC article.
-
[Quercetin improves pulmonary arterial hypertension in rats by regulating the HMGB1/RAGE/NF-κB pathway].Nan Fang Yi Ke Da Xue Xue Bao. 2023 Sep 20;43(9):1606-1612. doi: 10.12122/j.issn.1673-4254.2023.09.19. Nan Fang Yi Ke Da Xue Xue Bao. 2023. PMID: 37814876 Free PMC article. Chinese.
-
Identification of biomarkers, pathways, and potential therapeutic targets for heart failure using next-generation sequencing data and bioinformatics analysis.Ther Adv Cardiovasc Dis. 2023 Jan-Dec;17:17539447231168471. doi: 10.1177/17539447231168471. Ther Adv Cardiovasc Dis. 2023. PMID: 37092838 Free PMC article.
-
COVID-19 Salivary Protein Profile: Unravelling Molecular Aspects of SARS-CoV-2 Infection.J Clin Med. 2022 Sep 22;11(19):5571. doi: 10.3390/jcm11195571. J Clin Med. 2022. PMID: 36233441 Free PMC article.
References
-
- Adhikari N, Basi DL, Carlson M, Mariash A, Hong Z, Lehman U, Mullegama S, Weir EK, Hall JL. Increase in GLUT1 in smooth muscle alters vascular contractility and increases inflammation in response to vascular injury. Arterioscler Thromb Vasc Biol 31: 86–94, 2011. doi:10.1161/ATVBAHA.110.215004. - DOI - PMC - PubMed
Publication types
MeSH terms
Substances
Grants and funding
LinkOut - more resources
Full Text Sources
Other Literature Sources
Medical
Molecular Biology Databases