Age reprogramming and epigenetic rejuvenation
- PMID: 30572909
- PMCID: PMC6300877
- DOI: 10.1186/s13072-018-0244-7
Age reprogramming and epigenetic rejuvenation
Abstract
Age reprogramming represents a novel method for generating patient-specific tissues for transplantation. It bypasses the de-differentiation/redifferentiation cycle that is characteristic of the induced pluripotent stem (iPS) and nuclear transfer-embryonic stem (NT-ES) cell technologies that drive current interest in regenerative medicine. Despite the obvious potential of iPS and NT-ES cell-based therapies, there are several problems that must be overcome before these therapies are safe and routine. As an alternative, age reprogramming aims to rejuvenate the specialized functions of an old cell without de-differentiation; age reprogramming does not require developmental reprogramming through an embryonic stage, unlike the iPS and NT-ES cell-based therapies. Tests of age reprogramming have largely focused on one aspect, the epigenome. Epigenetic rejuvenation has been achieved in vitro in the absence of de-differentiation using iPS cell reprogramming factors. Studies on the dynamics of epigenetic age (eAge) reprogramming have demonstrated that the separation of eAge from developmental reprogramming can be explained largely by their different kinetics. Age reprogramming has also been achieved in vivo and shown to increase lifespan in a premature ageing mouse model. We conclude that age and developmental reprogramming can be disentangled and regulated independently in vitro and in vivo.
Keywords: Age reprogramming; Epigenetic clock; Epigenetic rejuvenation; Reprogramming factors; Somatic cell nuclear transfer (SCNT); eAge; iPS cells.
Figures
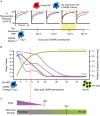
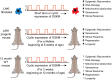
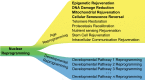
Similar articles
-
Deconstructing age reprogramming.J Biosci. 2019 Sep;44(4):106. J Biosci. 2019. PMID: 31502583 Review.
-
Cell reprogramming: Therapeutic potential and the promise of rejuvenation for the aging brain.Ageing Res Rev. 2017 Nov;40:168-181. doi: 10.1016/j.arr.2017.09.002. Epub 2017 Sep 10. Ageing Res Rev. 2017. PMID: 28903069 Review.
-
Rejuvenation by Partial Reprogramming of the Epigenome.Rejuvenation Res. 2017 Apr;20(2):146-150. doi: 10.1089/rej.2017.1958. Rejuvenation Res. 2017. PMID: 28314379 Review.
-
The role of the reprogramming method and pluripotency state in gamete differentiation from patient-specific human pluripotent stem cells.Mol Hum Reprod. 2018 Apr 1;24(4):173-184. doi: 10.1093/molehr/gay007. Mol Hum Reprod. 2018. PMID: 29471503
-
Epigenetic rejuvenation.Genes Cells. 2012 May;17(5):337-43. doi: 10.1111/j.1365-2443.2012.01595.x. Epub 2012 Apr 4. Genes Cells. 2012. PMID: 22487104 Free PMC article. Review.
Cited by
-
Deconstructing age reprogramming.J Biosci. 2019 Sep;44(4):106. J Biosci. 2019. PMID: 31502583 Review.
-
Opportunities and Challenges in Stem Cell Aging.Adv Exp Med Biol. 2021;1341:143-175. doi: 10.1007/5584_2021_624. Adv Exp Med Biol. 2021. PMID: 33748933 Review.
-
Restoring aged stem cell functionality: Current progress and future directions.Stem Cells. 2020 Sep;38(9):1060-1077. doi: 10.1002/stem.3234. Epub 2020 Jun 18. Stem Cells. 2020. PMID: 32473067 Free PMC article. Review.
-
β-hydroxybutyrate and its metabolic effects on age-associated pathology.Exp Mol Med. 2020 Apr;52(4):548-555. doi: 10.1038/s12276-020-0415-z. Epub 2020 Apr 8. Exp Mol Med. 2020. PMID: 32269287 Free PMC article. Review.
-
Initiation phase cellular reprogramming ameliorates DNA damage in the ERCC1 mouse model of premature aging.Front Aging. 2024 Jan 23;4:1323194. doi: 10.3389/fragi.2023.1323194. eCollection 2023. Front Aging. 2024. PMID: 38322248 Free PMC article.
References
-
- Wakayama S, Mizutani E, Wakayama T. Production of cloned mice from somatic cells, ES cells, and frozen bodies. In: Wassarman PM, Soriano PM, editors. Methods in enzymology. Cambridge: Academic Press; 2010. pp. 151–169. - PubMed
Publication types
MeSH terms
LinkOut - more resources
Full Text Sources
Other Literature Sources
Medical
Research Materials